6.4: The historical record of plate interactions
- Page ID
- 22635
\( \newcommand{\vecs}[1]{\overset { \scriptstyle \rightharpoonup} {\mathbf{#1}} } \)
\( \newcommand{\vecd}[1]{\overset{-\!-\!\rightharpoonup}{\vphantom{a}\smash {#1}}} \)
\( \newcommand{\id}{\mathrm{id}}\) \( \newcommand{\Span}{\mathrm{span}}\)
( \newcommand{\kernel}{\mathrm{null}\,}\) \( \newcommand{\range}{\mathrm{range}\,}\)
\( \newcommand{\RealPart}{\mathrm{Re}}\) \( \newcommand{\ImaginaryPart}{\mathrm{Im}}\)
\( \newcommand{\Argument}{\mathrm{Arg}}\) \( \newcommand{\norm}[1]{\| #1 \|}\)
\( \newcommand{\inner}[2]{\langle #1, #2 \rangle}\)
\( \newcommand{\Span}{\mathrm{span}}\)
\( \newcommand{\id}{\mathrm{id}}\)
\( \newcommand{\Span}{\mathrm{span}}\)
\( \newcommand{\kernel}{\mathrm{null}\,}\)
\( \newcommand{\range}{\mathrm{range}\,}\)
\( \newcommand{\RealPart}{\mathrm{Re}}\)
\( \newcommand{\ImaginaryPart}{\mathrm{Im}}\)
\( \newcommand{\Argument}{\mathrm{Arg}}\)
\( \newcommand{\norm}[1]{\| #1 \|}\)
\( \newcommand{\inner}[2]{\langle #1, #2 \rangle}\)
\( \newcommand{\Span}{\mathrm{span}}\) \( \newcommand{\AA}{\unicode[.8,0]{x212B}}\)
\( \newcommand{\vectorA}[1]{\vec{#1}} % arrow\)
\( \newcommand{\vectorAt}[1]{\vec{\text{#1}}} % arrow\)
\( \newcommand{\vectorB}[1]{\overset { \scriptstyle \rightharpoonup} {\mathbf{#1}} } \)
\( \newcommand{\vectorC}[1]{\textbf{#1}} \)
\( \newcommand{\vectorD}[1]{\overrightarrow{#1}} \)
\( \newcommand{\vectorDt}[1]{\overrightarrow{\text{#1}}} \)
\( \newcommand{\vectE}[1]{\overset{-\!-\!\rightharpoonup}{\vphantom{a}\smash{\mathbf {#1}}}} \)
\( \newcommand{\vecs}[1]{\overset { \scriptstyle \rightharpoonup} {\mathbf{#1}} } \)
\( \newcommand{\vecd}[1]{\overset{-\!-\!\rightharpoonup}{\vphantom{a}\smash {#1}}} \)
When plates interact in different ways (convergent, divergent, etc.), various phenomena result. Some of these phenomena are short-lived and thus unlikely to end up preserved in the “deep time” geologic record (offset streams at transform boundaries, for instance). Others create more durable signatures that can be identified in rock millions and billions of years later (such as metamorphic belts of a consistent age, or sedimentary basins that record rifting). When a region experiences divergent tectonics, it forms one set of geological signatures, while a completely different set of rocks, structures, and topography forms as a result of subduction tectonics.
Mountain belts
Convergent plate boundaries between blocks of continental lithosphere are recorded in the geologic record through the construction of mountain belts. When fresh, these mountain belts are mountainous: that is, they show high relief landscapes that occur in linear belts, like the modern Alps or Himalayas. However, intense topographic relief is unlikely to persist in the geologic record. Over time, mountains are worn down by erosion: between landslides, glaciers, and rivers, once-lofty rock is taken downhill, reducing the overall topographic relief.

However, the signatures of mountain-building at the roots of those same mountains may be preserved. These include (1) folded and thrust-faulted sedimentary strata on the flanks of the mountain-belt and (2) foliated metamorphic rocks in the core of the mountain belt. If orogenic temperatures were high enough, some of the minerals in some of the metamorphic rock may melt, forming migmatite. Sufficient partial melting will generate (3) bodies of felsic magma that can cool to form granite plutons. These durable geologic signatures show us where the mountains used to be, even after the mountains themselves are gone.

Additionally, consider the situation from the perspective of the stuff that’s removed: the sediment generated by the erosion of the mountains has to go someplace. It goes downhill, and ends up deposited somewhere else, perhaps nearby or perhaps far away, but it adds clastic sediment to the geologic record somewhere adjacent to the mountain belt. A shift in the sedimentary character of a given stratigraphic column from carbonate to clastic can signal an orogeny turning on. Because these clastic strata can contain fossils, we can use their age to determine the timing of mountain-building. A subsequent reversion to carbonate sedimentation thereafter will be a signal that the mountains have been removed as a source of sediment.
Marine sediment shed off a neighboring mountain belt is collectively dubbed flysch. It consists of black shale, bentonite, graywacke, and conglomerate. Terrestrial sediment shed off a neighboring mountain belt is collectively dubbed molasse. Typically it consists of red shale, submature river sandstones, and conglomerate. Before early geologists had come to terms with the non-intuitive ways that metamorphic and plutonic rocks formed, these clastic strata were the first recognized signals of ancient mountain building. The key recognition here is that the bajillions of particles that make up clastic strata represent a tremendous amount of eroded source rock, a huge volume equivalent to vanished mountain ranges.
Cratons and the accretionary growth of continents
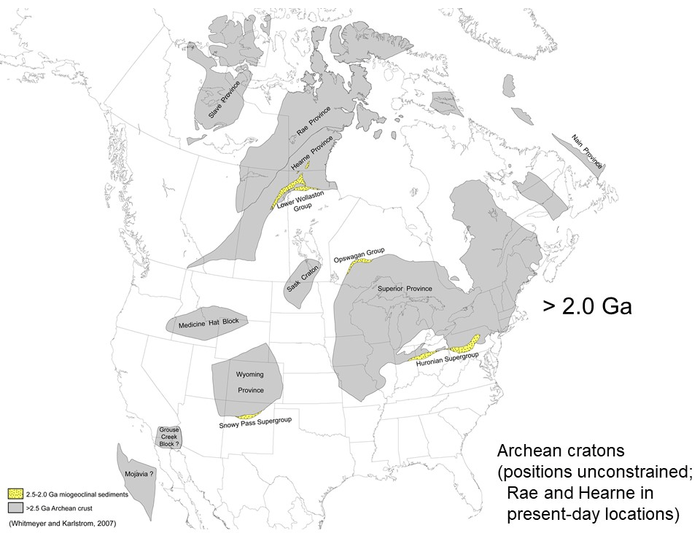
Over time, collisional events between masses of continental crust cause small blobs of crust to glom together and create larger, composite blobs of crust. This map shows the age of the various blocks of crust that make up the North American continent. If the crustal components are old, we tend to call them “cratons,” but if they are more recently tacked on, the preferred term is “terrane.” Whatever the name, the pattern is plain: the oldest bits of crust are surrounded by younger bits of crust. Continents start off relatively small, as resistant blobs of low-density material that survive subsequent collisions with neighboring blobs. Through convergence, they are sutured together and stay together. Seafloor sediment, volcanic island arcs, and other small masses of continental crust merge and stick: continents grow through the accretion of new terranes on their margins.
Ophiolites

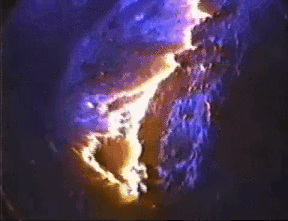
Earlier, we described the structure of the oceanic lithosphere as it forms through seafloor spreading at a divergent boundary. From bottom to top, it consists of mantle peridotite, gabbro, sheeted dikes of basalt, pillow basalt, and that may be topped with deep sea sediments, such as chert or shale. This sequence is both the structure of the oceanic crust as well as the structure of small slivers of rock we often find between accreted terranes. In other words, on dry land, in the heart of mountain belts, we often find what appear to be scraps of oceanic crust. These are called ophiolites. Before the advent of plate tectonics, it was difficult to explain ophiolites, but now we can recognize them as remnant shreds of the oceanic lithosphere that used to separate the two terranes – the scant remains of an ocean basin that was otherwise mostly destroyed through the process of subduction.
For instance, at Gros Morne National Park in Newfoundland, Canada, there is a huge area of exposed peridotite. Though the fresh peridotite would be green because of its olivine content, the wet climate of Newfoundland has rusted it all at Earth’s surface, making the landscape surface orange. Adjacent to the peridotite is layered gabbro, and adjacent to that are sheeted dikes and pillow basalts.
The record of subduction
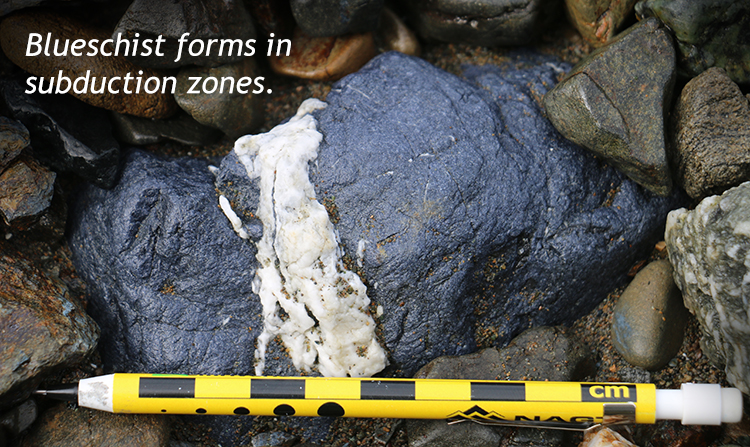
Subduction is a key component of plate tectonics. To recognize places where subduction has occurred in the geologic past, we look for metamorphic, igneous, and structural signatures. One of the basic aspects of modern subduction is the parallelism of oceanic trench and volcanic arc. These two settings have different blends of temperature and pressure, resulting in different “flavors” of metamorphic rocks. The trench and Wadati-Benioff zone will result in high pressure + low temperature metamorphic rocks, while the neighboring magmatic arc will produce moderate pressure + high temperature metamorphic rocks. These “paired metamorphic belts” allow us to distinguish the earliest records of subduction from the geologic record, and the rather different tectonic situation that preceded subduction during the Archean.
Few places preserve this better than coastal northern California. The rock there formed (and deformed) during the Mesozoic subduction of the Farallon Plate beneath the western North American Plate.
Did I Get It? - Quiz
Interpret the following geological characteristics as a plate boundary setting: blueschist, accretionary wedge, thrust faults.
a. Transform
b. Convergent (mountain-building)
c. Divergent (continental rifting)
d. Convergent (subduction)
e. Divergent (seafloor spreading)
- Answer
-
d. Convergent (subduction)
Interpret the following geological characteristics as a plate boundary setting: mafic volcanism, immature clastic sediments, normal faults.
a. Convergent (mountain-building)
b. Convergent (subduction)
c. Divergent (rift valley in continental crust)
d. Transform
e. Divergent (seafloor spreading)
- Answer
-
c. Divergent (rift valley in continental crust)
Interpret the following geological characteristics as a plate boundary setting: foliated metamorphic rocks, granite plutons, folding, ophiolites.
a. Divergent (seafloor spreading)
b. Convergent (subduction)
c. Divergent (continental rifting)
d. Convergent (mountain-building)
e. Transform
- Answer
-
d. Convergent (mountain-building)
Continents get larger through time due to ___________.
a. docking of cratons or accretion of terranes
b. rifting
c. eruption of pillow basalts
d. erosion
- Answer
-
a. docking of cratons or accretion of terranes
Ophiolites represent what process(es)?
a. Seafloor spreading followed by mountain-building
b. Left-lateral transform faulting
c. Subduction
d. Seafloor spreading
e. Mountain-building
- Answer
-
a. Seafloor spreading followed by mountain-building
Sedimentary basins
An important consequence of plate tectonics for Historical Geology is how the different plate boundary settings give rise to circumstances where sediments can accumulate and be preserved over the geological long term. Substantial loci of sedimentary deposition are referred to as basins.
Transform
Because transform plate boundaries largely conserve the land surface as it is, merely offsetting it in opposite directions, they do not tend to be exceptionally good basin formers. However, where there are small “jogs” in the orientation of the fault trace, transtension can open up relatively small wrench basins.
WRENCH BASINS
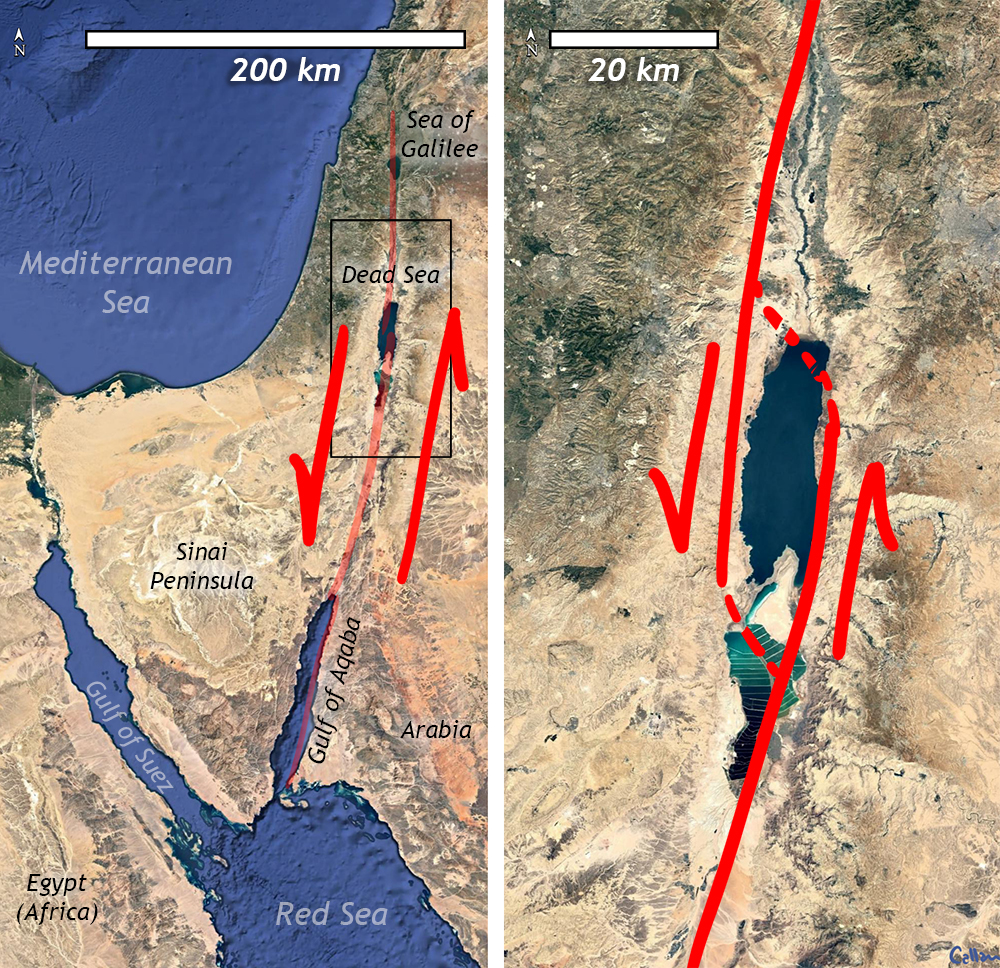
Wrench basins form when a right-lateral fault steps over to the right, or a left-lateral fault steps over to the left. The land between the end of one fault segment and the beginning of the next will sag. As we saw above, on a small scale this can generate a sag pond (or a low spot in a parking lot!), but on a larger scale it can produce substantial basins: these catchments dilate and create a topographic low spot into which sediment can pour from the surrounding highlands.
The Dead Sea, on the boundary between Israel, the West Bank, and Jordan, is an excellent modern example. Here, the Dead Sea Transform Fault, a left-lateral series of faults between the Sinai Peninsula and the Arabia Plate. As at Aqaba, and the Sea of Galilee, left steps in the fault system have stretched open low spots in the landscape, filled by water. At Aqaba, it’s an offshoot of the Red Sea. The Sea of Galilee is landlocked, and filled with freshwater.
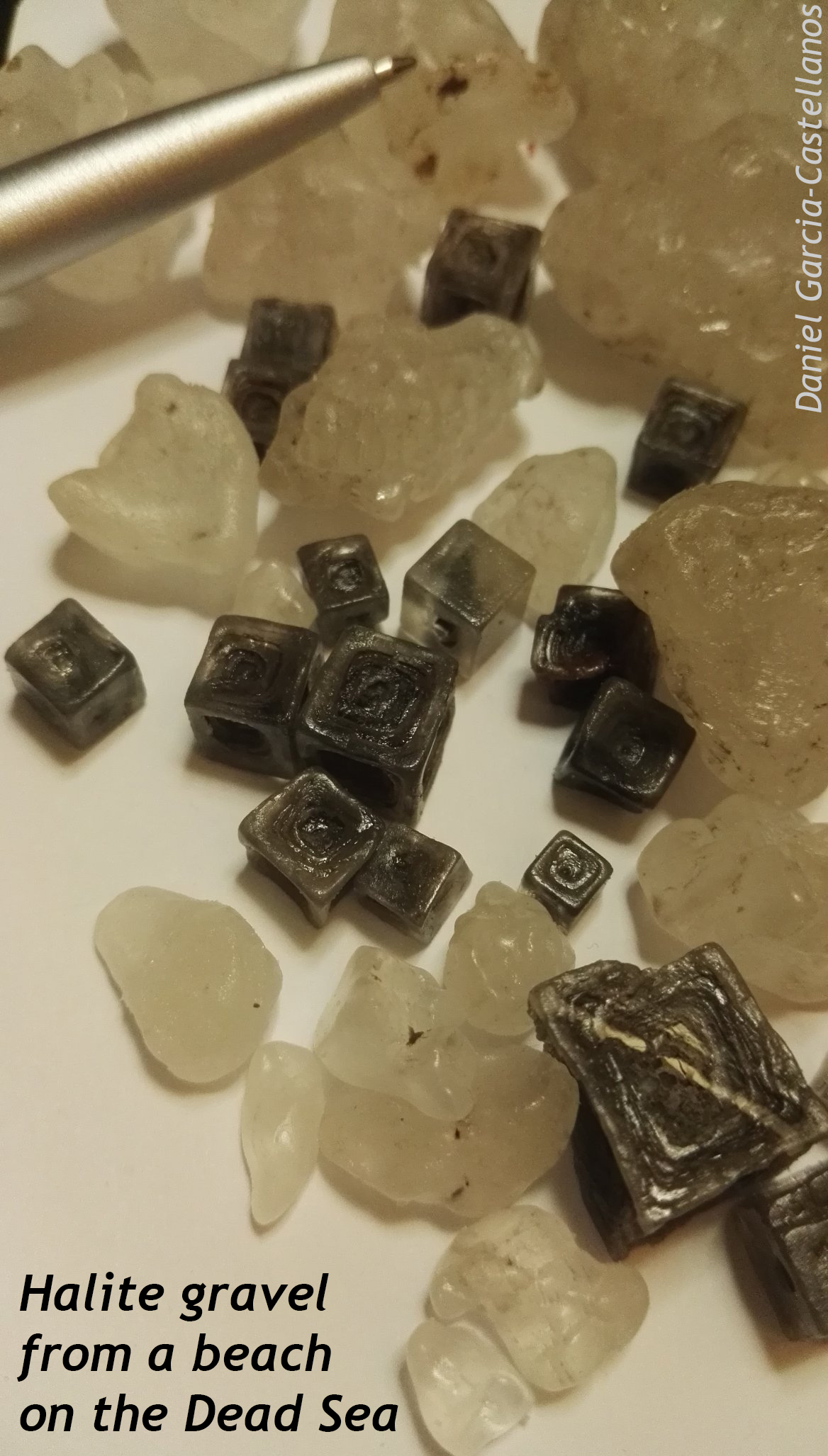
The Dead Sea is the lowest spot on land in the entire world. (There are of course, deeper spots in the ocean basins, such as the Marianas Trench.) The hot, dry climate and lack of replenishing water (especially in recent years) have resulted in an extremely salty body of water occupying the basin, currently at 430.5 meters below sea level (-1,412 feet in elevation), but dropping further every year.
Other modern examples include the Salton Sea (on the San Andreas Fault) and the Sea of Marmara (south of İstanbul on the North Anatolian Fault).
Wrench basins are not just a modern phenomenon, however. In true uniformitarian spirit, we find many preserved in numerous contexts in the geologic record. They can serve as excellent repositories of local lithologic diversity in situations where the source rocks have been completely eroded away.

For instance, in the heart of the Superior Craton of North American, there are a series of wrench basins that captured cobbles derived from the surrounding highlands: mountains that haven’t seen topographic relief since Archean times. Thanks to the preservation of these wrench basin conglomerates, we can see not only which areas were made low by tectonics, but what composition rocks made up the surrounding high country.
Divergent

The principal variety of basin that forms at a divergent setting is a rift basin. When rift basins are fresh, they accumulate immature clastic sediment. Over time, especially in large rift basins, the sediments may mature towards a higher proportion of quartz and clay. Some even see limestones at positions far removed from the basin margins. Volcanism is important too: as the crust stretches in a rift, it lowers the pressure on the underlying mantle. Decompression melting follows, which produces mafic magma. Eruption of basalt and intrusion of gabbro and diabase are common igneous features of rift basins.
RIFT BASINS
As we’ve already mentioned, the Great Rift Valley system of east Africa offers an outstanding modern example of a rift basin. Because of its location and timing, this is *the* place to look for fossils of human ancestors. Other modern examples of rift basins include Lake Baikal in Siberia (and a smaller equivalent to the south in Mongolia, Lake Khovsgol). The valley of Þingvellir (“Thingvellir”) in southwest Iceland, is celebrated by Icelanders as the seat of the world’s oldest functioning democracy, but geologists see it in a different light: it is a place where the Mid-Atlantic Ridge can be seen above sea level. All of these basins are bound by normal faults.
A fine example of an ancient rift basin can be found in central Virginia, where the Culpeper Basin is the largest of a dozen basins that preserve terrestrial sediments (and mafic igneous rocks) related to the break-up of the supercontinent Pangaea and the initial opening of the Atlantic Ocean.

The Culpeper Basin is filled with clastic sediment and mafic igneous rock. It shows coarsest-grained sediment at the margin basins. These alluvial fan deposits are different in composition in the west versus the east, as the alluvial fans in the two locations were draining highlands of very different compositions. In the west, limestone and basalt clasts predominate. (One of these western fanglomerates, the Leesburg Conglomerate, makes up the distinctive columns of the Hall of Statuary in the United States Capitol building.) In the east, the large clasts are mainly foliated metamorphic rocks derived from the neighboring Piedmont geologic province. Further from the basin margins, the sediments get finer. They include arkosic sands and mud deposits.
Lake deposits from the central Culpeper Basin have been contact metamorphosed to yield layers of multicolored hornfels that produce surprisingly durable cliffs:
The Culpeper Basin is one of many basins that formed in the heart of Pangaea during its breakup. Some of these basins connected up — a line of “weakest links” — and ripped open to make the Atlantic Ocean. Basins east of that line are now in Africa and Europe. Basins west of that line, including the Culpeper Basin, are called the “Newark Supergroup” basins. Other examples in this collection include the Gettysburg Basin, the eponymous Newark Basin, the Hartford Basin of Connecticut, and Canada’s Bay of Fundy. Collectively, the Newark Supergroup basins are quite extensive.
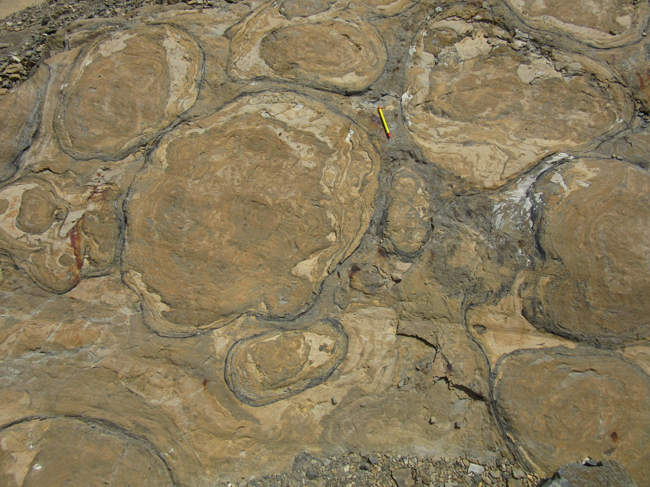
Another huge rift basin can be found in northwestern Montana and adjacent states and territories: the Belt Supergroup was deposited in the Belt Basin in Mesoproterozoic sedimentary time. Like the Culpeper Basin, it’s coarsest at the edges and at the bottom. It’s also similar in having intercalated mafic igneous rocks. It’s different in being much larger, and lasting long enough to develop more mature sediments, including thick carbonate packages within its stratigraphic sequence.

Another ancient rift basin worth noting is the Mid-Continent Rift of North America (also called the Keweenawan), which extends from Lake Superior in Minnesota to the southwest, diving beneath sedimentary cover for much its length (but still detectable via its distinctive positive gravity anomaly signature). This gigantic rift system developed in the Mesoproterozoic immediately before the Grenville Orogeny.
Convergent
Subduction at convergent plate boundaries can also induce sedimentary basins to form. There are three principal varieties to consider: foreland basins, forearc basins, and backarc basins.
FORELAND BASINS
Foreland basins form on the continental crust as subduction loads the crust with a heavy mountain range. Thrust faulting thickens the crust adjacent to a subduction zone, and this additional rock mass causes the adjacent crust to sag. The sagging creates accommodation space for sediment.
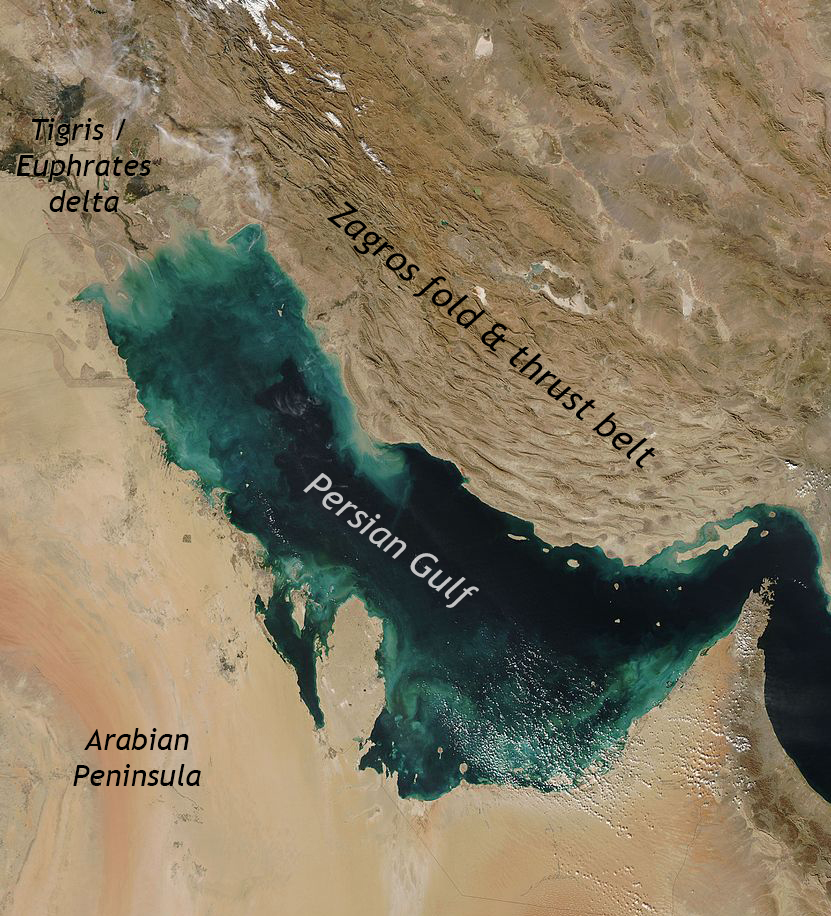
A modern foreland basin can be seen in the Persian Gulf. The Persian Gulf is a foreland basin between the Arabian Peninsula and the southern edge of Eurasia: Iran’s Zagros Mountains. As the Arabian Plate and the Eurasian Plate converge, thrust-loading of the crust in the Zagros has caused the adjacent crust to sag and make the low spot of the Persian Gulf.
An Ordovician example can be found in the mid-Atlantic region: the Queenston clastic wedge is a thick package of sediment including both marine flysch and terrestrial molasse that formed in response to downward flexure of the crust induced by the Taconian Orogeny. The same pattern repeated during the Devonian Acadian Orogeny: the Catskill clastic wedge is the name given to this subsequent foreland basin, partially overlapping in space the region occupied by its Ordovician predecessor.

In Cretaceous western North America, another foreland basin formed: the Western Interior Seaway stretched from the Gulf of Mexico to the Arctic Ocean. Seawater flooded a vast trough of land that sagged downward as subduction of the Farallon Plate caused the Sevier Orogeny. This episode of thin-skinned deformation thrust thick slices of sedimentary rock into towering peaks.
FOREARC BASINS
Forearc basins form on the trench side of volcanic arcs. Subduction at the trench keeps the crust low, and the neighboring volcanic arc sheds copious sediment that can fill the basin.

A classic example is the Great Central Valley of California, which is a forearc basin of Jurassic/Cretaceous age. At that time, subduction of the Farallon Plate generated a thick accretionary wedge of seafloor rocks. This is preserved in the modern day as the bulk of California’s Coast Ranges. Inland, this same subduction manifested as a vibrant volcanic arc, the roots of which are today preserved as the Sierra Nevada batholith, the voluminous wad of granites that can be found in places such as Yosemite National Park. During the Cretaceous, the Sierra Nevada would have looked much like the modern Andes Mountains of South America. Though the volcanoes themselves have since been eroded away, the extent of their source magma chambers is gloriously revealed in the high country of that excellent mountain range.
Between the Coast Ranges’ accretionary wedge and the Sierra’s magmatic arc is the vast, hyperflat Great Central Valley. Though the valley is a modern topographic low, it corresponds very closely in areal extent with the Cretaceous submarine forearc basin:
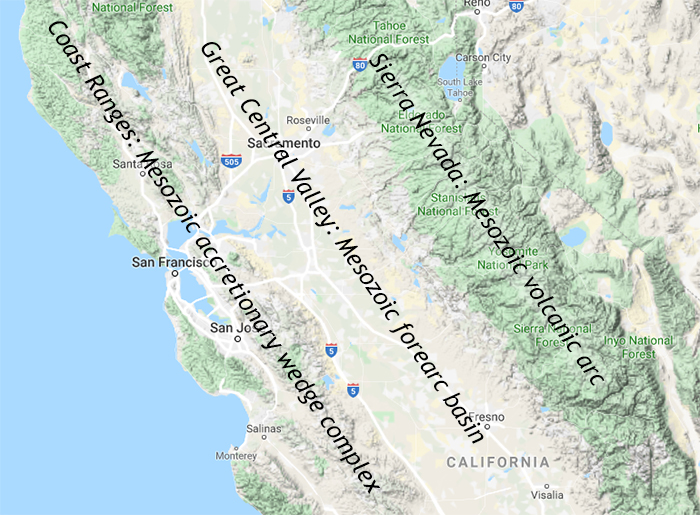
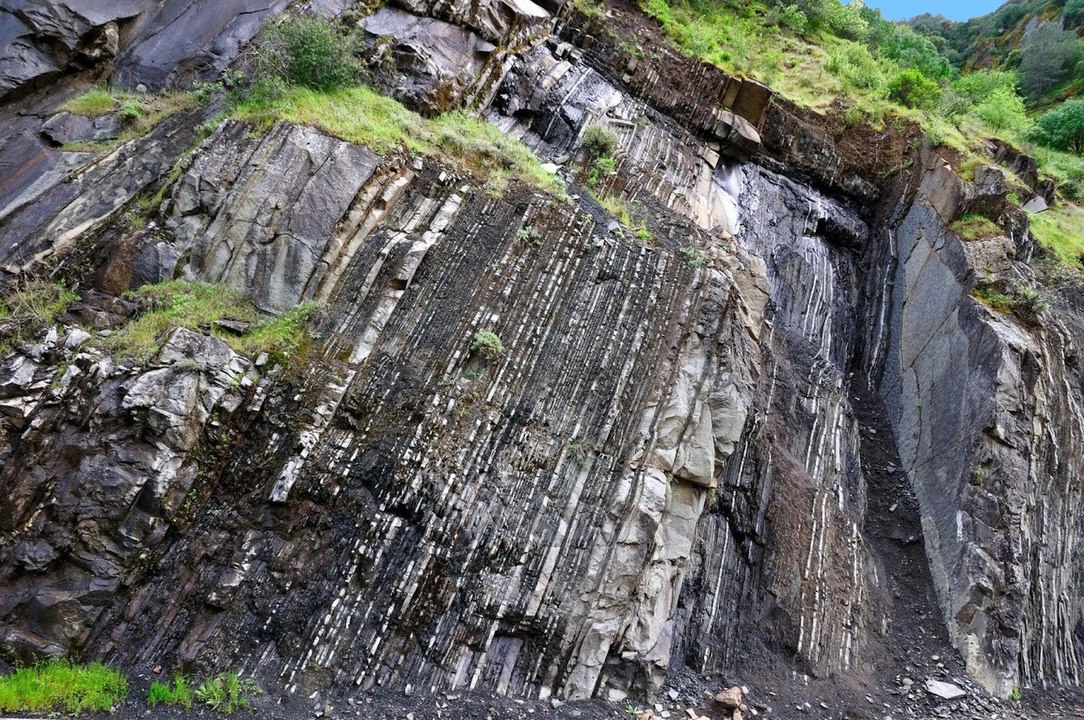
The Great Central Valley is 60 to 100 km (40 to 60 miles) wide. It stretches approximately 720 km (450 miles) along a north-northwest to south-southeast trend, paralleling the volcanic arc to the east and the accretionary wedge to the west. Clastic sediments deposited in this basin make up the 12 km-thick (40,000’) Great Valley Sequence. Mostly shale, it also includes substantial bodies of sandstone and conglomerate that probably represent Mesozoic abyssal fan systems, sourced from the Sierra Nevada volcanic arc.
BACK-ARC BASINS
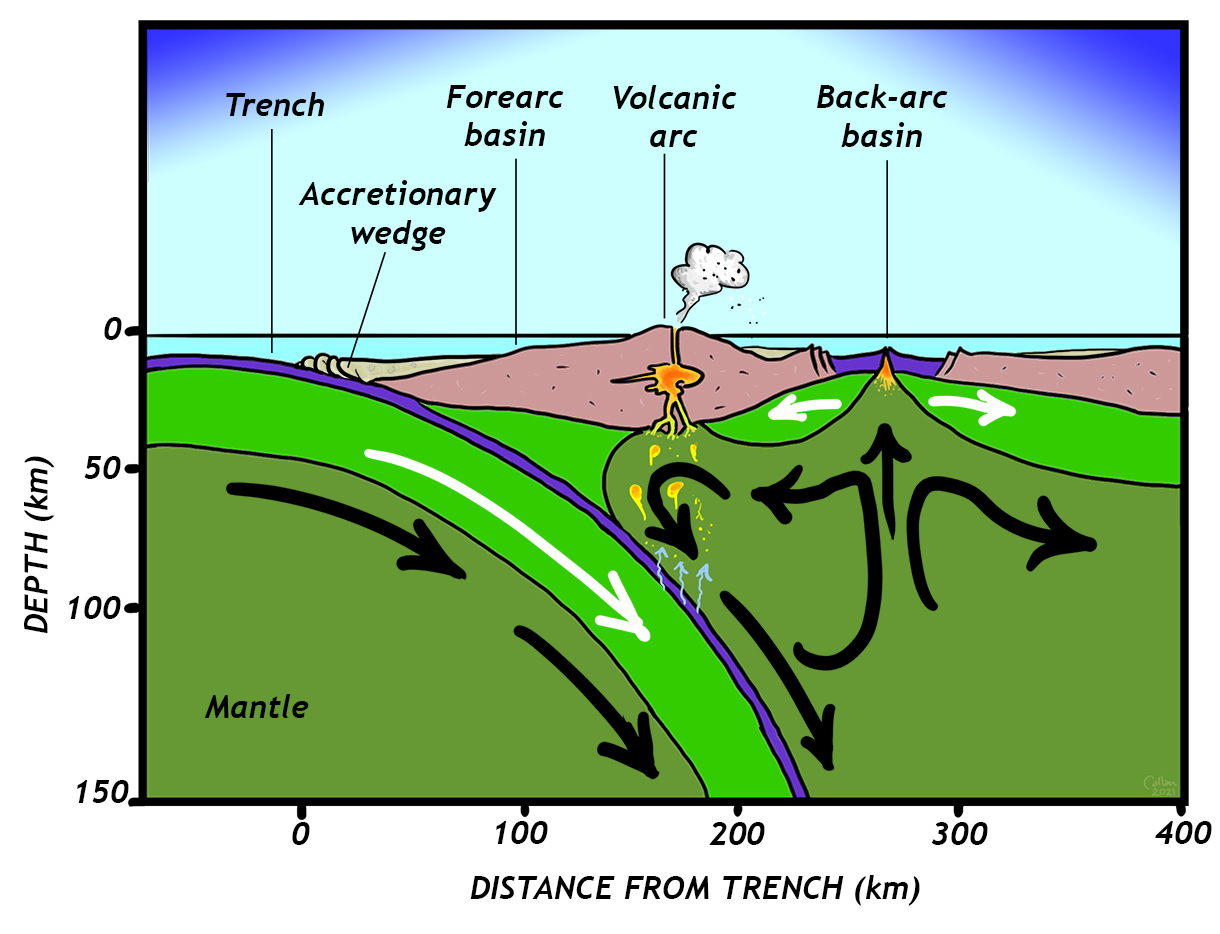
Back-arc basins are the final category associated with convergent boundaries. There’s a plot twist though: ironically, they actually require divergence to form! They result from tensional forces caused by rising mantle material coupled with oceanic trench rollback (the oceanic trench shifts over time in the direction of the source of the subducting plate). The arc crust is under extension or rifting as a result of the sinking of the subducting slab. A good modern example of back-arc spreading is the Sea of Japan, a modest ocean basin that has opened between the islands of Japan (a volcanic arc) and the Asian mainland.
Did I Get It? - Quiz
Transform boundaries can produce what kind of basin?
a. Foreland basin
b. Wrench basin
c. Rift basin
d. Back-arc basin
- Answer
-
b. Wrench basin
What kind of basin forms as a result of "loading" the crust with a thick mountain belt due to thrust faulting?
a. Foreland basin
b. Forearc basin
c. Wrench basin
d. Back-arc basin
- Answer
-
b. Foreland basin
The Persian Gulf is what kind of basin?
a. Wrench basin
b. Foreland basin
c. Rift basin
d. Forearc basin
e. Back-arc basin
- Answer
-
b. Foreland basin
What kind of basin is shown?
a. Wrench basin
b. Forearc basin
c. Back-arc basin
d. Rift basin
e. Foreland basin
- Answer
-
d. Rift basin
A modern-day example of a back-arc basin is ___________.
a. central Virginia
b. the Wasatch front of Utah
c. east Africa
d. the Mid-Atlantic Ridge
e. west of Japan
- Answer
-
e. west of Japan
What sort of basin formed in the middle of the ancestral North American continent during the Mesoproterozoic Eon? (near the western Great Lakes region)
a. Wrench basin
b. Foreland basin
c. Rift basin
d. Forearc basin
- Answer
-
c. Rift basin
What sort of basin formed in the middle of North America during the Cretaceous, running from the Arctic Ocean south to the Gulf of Mexico?
a. Wrench basin
b. Foreland basin
c. Back-arc basin
- Answer
-
b. Foreland basin