16.3: Evolution
- Page ID
- 9633
16.3.1. Requirements for Cyclogenesis
Seven conditions are necessary for tropical cyclones to form: a warm sea surface, non-zero Coriolis force, nonlocal conditional instability, high humidity in the mid troposphere, weak ambient wind shear, enhanced synoptic-scale vorticity, and a trigger.
16.3.1.1. Warm Sea Surface
The sea surface temperature (SST) must be approximately 26.5°C or warmer (Figs. 16.8 and 16.9 on this page, and 16.51 at the end of this chapter), and the warm surface waters must be at least 50 m deep. This warm temperature is needed to enable strong evaporation and heat transfer from the sea surface into the boundary layer. The warmer, more-humid boundary-layer air serves as the fuel for thunderstorms in the tropical cyclone.

The fast winds in tropical cyclones create large waves that stir the top part of the ocean. If the warm waters are too shallow, then this turbulent mixing will stir colder deeper water up to the surface (see INFO Box two pages later). When this happens, the sea-surface temperature decreases below the 26.5°C threshold, and the tropical cyclone kills itself.
16.3.1.2. Coriolis Force
Tropical cyclones cannot exist within about 500 km of the equator (i.e., ≤ 5° latitude), because Coriolis force is near zero there (and is exactly zero right at the equator). Rarely, very small-diameter tropical cyclones have been observed closer to the equator, but none are observed right at the equator. Tropical cyclones cannot form at the equator, and existing cyclones cannot cross the equator (Fig. 16.7).
Without Coriolis force, boundary-layer air would be sucked directly into the eye by the low pressure there. Thus, air molecules would accumulate in the eye, causing pressure to increase towards ambient values. The result is that the low pressure would disappear, winds would die, and the tropical cyclone would cease to exist in less than a day. This is what happens to tropical cyclones that approach the equator (Fig. 16.10).

But with Coriolis force, winds in the bottom half of the troposphere are forced around the eye at gradient- or cyclostrophic-wind speeds. Namely, most of the air is flowing tangentially around the eye rather than flowing radially into it. Only close to the ground does drag change the winds into boundarylayer gradient winds, resulting in a small amount of convergence toward the eye. Thus, the tropical cyclone can persist for many days.
16.3.1.3. Nonlocal Conditional Instability
Because tropical cyclones are made of thunderstorms, the tropical environment must have sufficient nonlocal conditional instability to support deep thunderstorm convection. Namely, there must be a stable layer (i.e., a cap) above a warm humid boundary layer, and the mid-troposphere must be relatively cool compared to the boundary layer.
The warm humid boundary layer is achieved via strong heat and moisture fluxes from the warm sea surface into the air. The cap is the trade-wind inversion that was discussed in the General Circulation chapter. These conditions, combined with a cool mid troposphere, lead to large values of convective available potential energy (CAPE), as was thoroughly explained in the Thunderstorm chapter. Hence, large values of CAPE imply sufficient nonlocal conditional instability for tropical cyclones.
16.3.1.4. High Humidity in Mid-troposphere
In a deep layer of air centered at roughly 5 km above sea level, humidity must be high. Otherwise, the incipient thunderstorms cannot continue to grow and organize into tropical cyclones.
Note that this differs from mid-latitude thunderstorms, where a drier mid-troposphere allows more violent thunderstorms. When dry environmental air is entrained into the sides of mid-latitude thunderstorms, some of the storm’s hydrometeors evaporate, causing the strong downdrafts that define supercell storms and which create downbursts, gust fronts, and can help trigger tornadoes.
In the tropics, such downbursts from any one thunderstorm can disrupt neighboring thunderstorms, reducing the chance that neighboring thunderstorms can work together in an incipient eyewall. Also, the cold downburst air accumulates at the bottom of the troposphere, thereby increasing static stability and reducing deep convection.
16.3.1.5. Weak Ambient Wind Shear
Wind shear within four degrees of latitude of the incipient storm must be weak (∆M < 10 m s–1 between pressure levels 80 and 25 kPa) to enable thunderstorm clusters to form. These clusters are the precursors to tropical cyclones.
If the shear is too strong, the updrafts in the thunderstorms become tilted, and latent heating due to water-vapor condensation is spread over a broader area. This results in less-concentrated warming, and a reduced ability to create a low-pressure center at sea level around which the thunderstorms can become organized into a tropical cyclone.
This requirement differs from that for mid-latitude thunderstorms. At mid-latitudes, strong shear in the ambient environment is good for storms because it encourages the creation of mesocyclones and supercell thunderstorms. Apparently, in the tropics such rotation of individual thunderstorms is bad because it interferes with the collaboration of many thunderstorms to create an eyewall.
16.3.1.6. Synoptic-scale Vorticity
A relative maximum of relative vorticity in the bottom half of the troposphere can help organize the thunderstorms into an incipient tropical cyclone. Otherwise, any thunderstorms that form would act somewhat independently of each other.
Near-surface winds cause net ocean transport 90° to the right of the wind vector in the N. Hemisphere, as explained later in the Storm Surge section. Given tangential winds that circle a tropical cyclone, we expect the net Ekman transport (average ocean currents induced by the tropical cyclone) to be outward (Fig. 16.a)
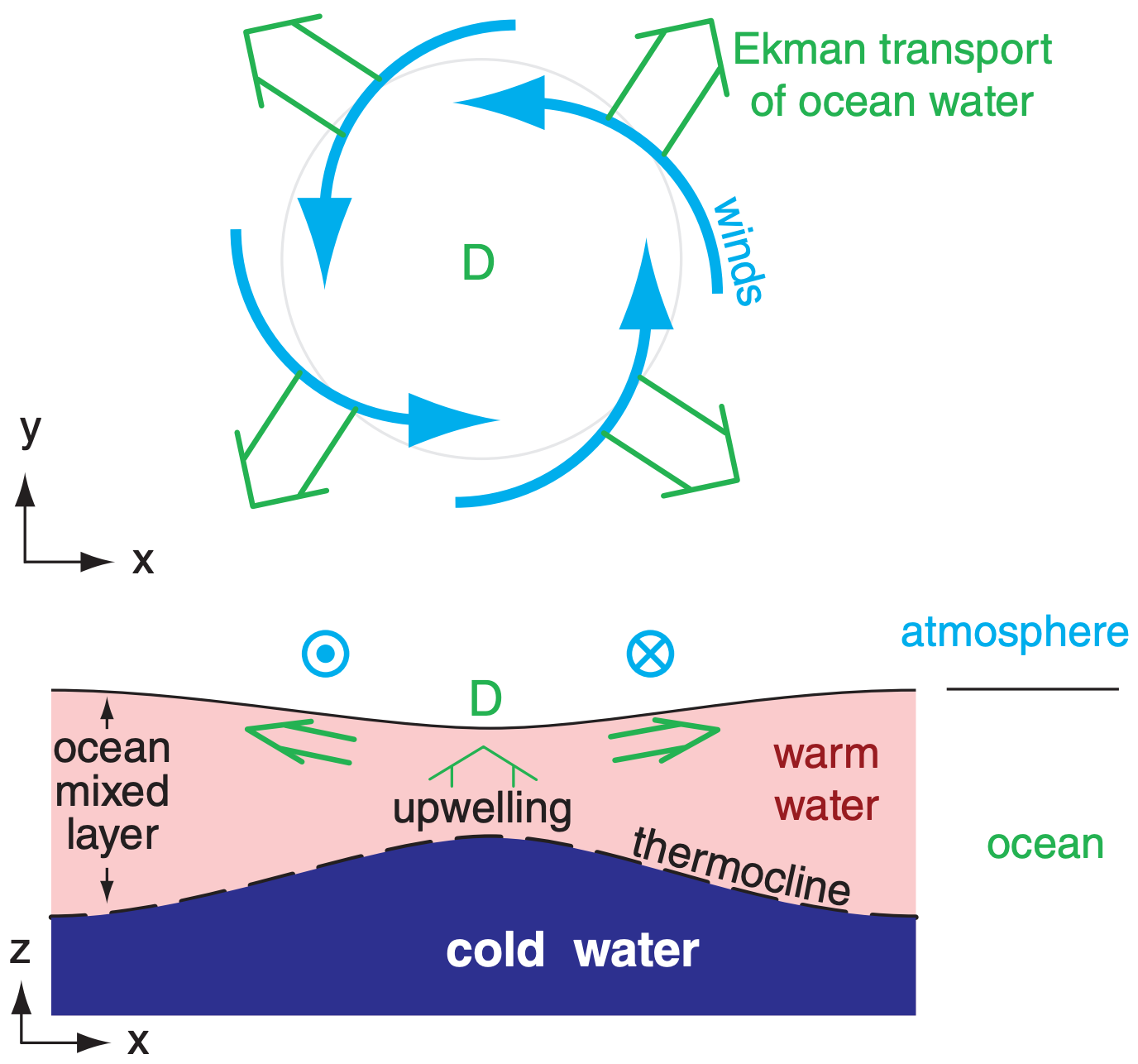
Such horizontal divergence (D) of the ocean-surface water does three things: (1) it lowers the sea surface by removing sea water; (2) it causes upwelling of water toward the surface; and (3) it causes the colder deep water to be brought closer to the surface (Fig. 16.a-bottom). The thermocline is the interface between cold deep water and warmer mixed-layer above.
The tropical cyclone brings cold water closer to the surface, where ocean mixed-layer turbulence caused by breaking waves can further mix the warm and cold waters. Thus, tropical cyclones will kill themselves by cooling the sea-surface temperature unless the prestorm warm ocean mixed-layer is ≥ 50 m deep.
16.3.2. Tropical Cyclone Triggers
Even if all six previous conditions are satisfied, a method to trigger the tropical cyclone is also needed. A trigger is anything that creates synoptic-scale horizontal convergence in the atmospheric boundary layer. Such horizontal convergence forces upward motion out of the boundary-layer top as required by mass conservation (see the Atmospheric Forces & Winds chapter). Synoptic-scale upward motion can initiate and support an organized cluster of thunderstorms — incipient tropical cyclones.
Some triggers are: the ITCZ, easterly waves, Monsoon troughs, mid-latitude fronts that reach the tropics, and Tropical Upper Tropospheric Troughs.
16.3.2.1. ITCZ
The Intertropical Convergence Zone (ITCZ, see General Circulation chapter) is the region where the northeasterly trade winds from the Northern Hemisphere meet the southeasterly trade winds from the Southern Hemisphere. This convergence zone shifts between about 10°N during August and September (end of N. Hemisphere summer, Fig. 16.11), and 10°S during February and March (end of S. Hemisphere summer). During these months of maximum asymmetry in global heating, the ITCZ is far enough from the equator for sufficient Coriolis force to enable tropical cyclones.
16.3.2.2. African Easterly Wave
Although equatorial Africa is hot, the Sahara Desert (roughly spanning 15° to 30°N) and Arabian Peninsula are even hotter. This temperature excess is within an atmospheric boundary layer that can reach 5 km depth by late afternoon, although average depth over 24 h is about 3 km. Thus, along the southern edge of the Sahara and the Arabian Peninsula is a strong temperature gradient (Fig. 16.12).

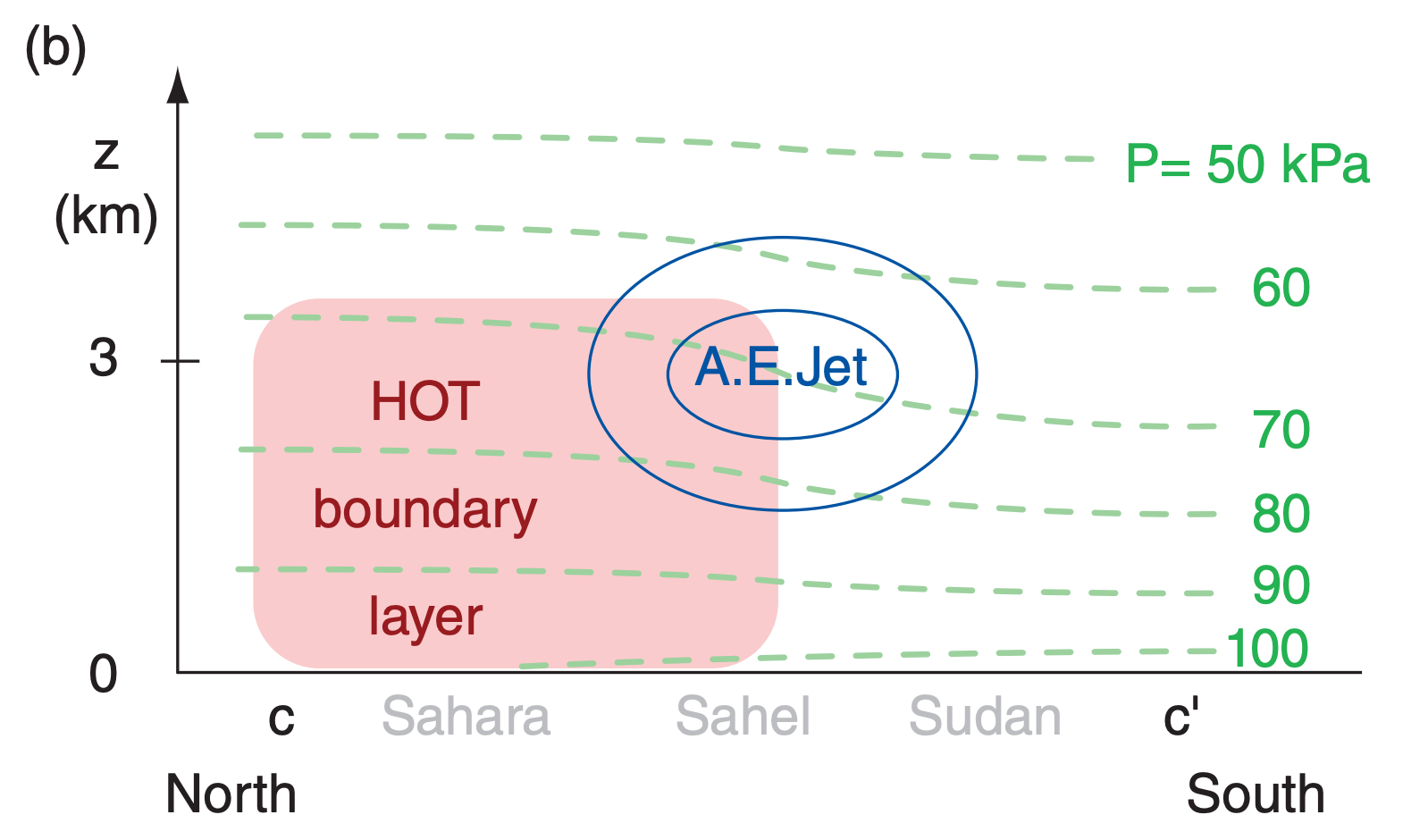
As explained in the thermal-wind section of the General Circulation chapter, a north-south temperature gradient creates a north-south pressure gradient that increases with altitude (up to the top of the boundary layer for this scenario). For Africa, higher pressure aloft is over the Sahara Desert and lower pressure aloft is further south over the Sudan and Congo.
This pressure gradient drives a geostrophic wind from the east over the African Sahel (at about 15°N). These winds reach maximum speeds of 10 to 25 m s–1 at an altitude of about 3 km in late summer. This is called the African Easterly Jet (AEJ).
Like the subtropical and mid-latitude jets, the AEJ is barotropically and baroclinically unstable. This means that the jet tends to meander north and south while blowing from east to west. The mountains in eastern Africa (Ethiopia Highlands and Darfur Mountains) can trigger such oscillations.
The oscillations in this low-altitude jet are called waves, and are known as Easterly Waves because the wave troughs and crests move from the east at speeds of 7 to 8 m s–1. These waves have wavelength of about 2,000 to 2,500 km, and have a wave period of 3 to 4 days. Although these waves are created over Africa, they continue to propagate west across the tropical North Atlantic, the Caribbean, central America, and finally into the tropical Northeast Pacific (Fig. 16.13).

Easterly waves can trigger tropical cyclones because of the low-altitude convergence that occurs east of the wave troughs. These waves are found predominantly in a latitude band between 10°N and 30°N, where there is lower pressure to the south and the subtropical high-pressure belt to the north. Thus, a trough of low pressure in an easterly wave corresponds to a region where the isobars meander to the north (Fig. 16.14) in the N. Hemisphere.
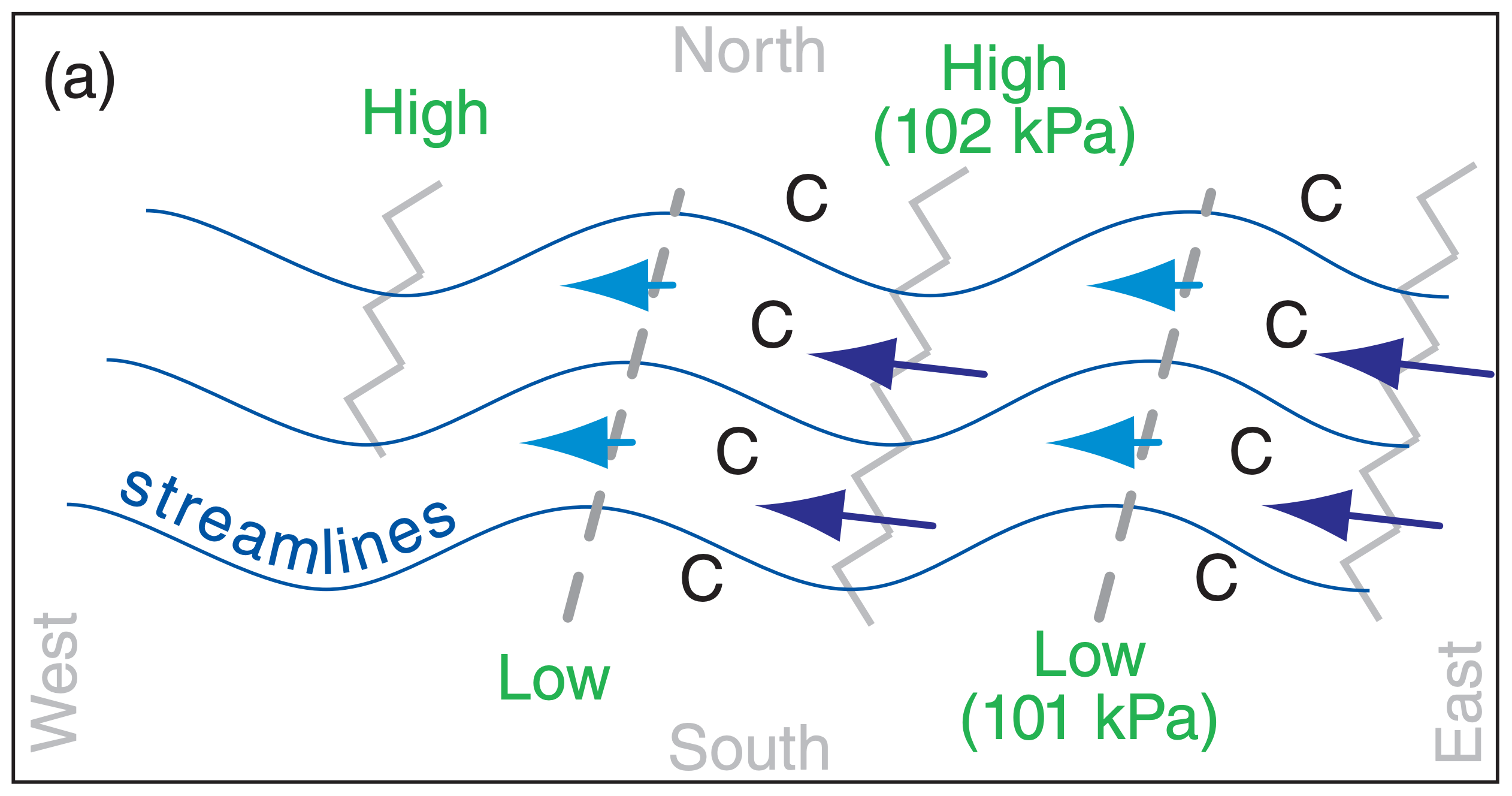

Because the pressure gradient is weak and sometimes difficult to analyze from sparsely spaced weather stations in this region, tropical meteorologists will often look at the streamlines instead of isobars. Streamlines are weather-map lines that are everywhere parallel to the flow at any instant in time (see the Regional Winds chapter). They more-or-less follow the isobars. Thus, regions where streamlines for low-altitude winds meander to the north can also be used to locate troughs in the easterly waves.
Recall that mid-latitude cyclones are favored in the updrafts under divergence regions in the polar jet (centered at the top of the troposphere). However, for tropical cyclogenesis we also look for updrafts above the convergence regions of the jet near the bottom of the troposphere (Fig. 16.15a).
Where are these convergence regions in easterly waves? According to the Atmospheric Forces & Winds chapter, winds are slower-than-geostrophic around troughs, and faster around ridges. This rule continues to hold in the tropics and subtropics. Thus, the convergence zone with fast inflow and slow outflow is east of the trough axis (Fig. 16.14a).
Also, since the trough axis often tilts eastward with increasing altitude, it means that a mid-tropospheric trough is often over a lower-tropospheric convergence region. This trough has the cyclonic vorticity that encourages organization of thunderstorms into incipient tropical cyclones.
It is these regions east of troughs in easterly waves where the thunderstorms of incipient tropical cyclones can be triggered (Fig. 16.14b). As the easterly waves propagate from east to west, so move the convergence regions favoring tropical cyclone formation. This convergence mechanism is important for tropical cyclone triggering in both the North Atlantic and Northeast Pacific. Roughly 85% of intense Atlantic hurricanes are triggered by easterly waves.
16.3.2.3. Monsoon Trough
A monsoon trough (MT) is where the ITCZ merges into a monsoon circulation. For example, Fig. 16.16 shows a monsoon low pressure (L) region over southeast Asia (left side of Fig.), with winds rotating counterclockwise around it. Near the dashed line labeled MT in the western Pacific, monsoon winds from the southwest converge with easterly trade winds from the Hawaiian (Pacific) High.

Thus, the monsoon trough in the western Pacific is a convergence region that can trigger thunderstorms for incipient typhoons. Similar monsoon troughs have been observed in the Indian Ocean and the Eastern Pacific. In Fig. 16.16, the region where the monsoon trough in the western Pacific meets the ITCZ has enhanced convergence, and is a more effective trigger for tropical cyclones.
16.3.2.4. Mid-latitude Frontal Boundary
Sometimes cold fronts from midlatitudes can move sufficiently far equatorward to reach the subtropics. Examples include cold fronts reaching southern Florida (Fig. 16.17). Although these fronts are often weak and slow moving by the time the reach the subtropics, they nonetheless have convergence across them that can trigger tropical cyclone thunderstorms in the Caribbean and the subtropical western North Atlantic.

16.3.2.5. TUTT
TUTT stands for Tropical Upper Tropospheric Trough. This is a high-altitude cold-core low-pressure system that can form in the subtropics. East of the TUTT axis is a region of horizontal divergence aloft, which creates upward motion below it in order to satisfy air mass continuity (Fig. 16.15b). This upward motion can help trigger thunderstorms as precursors to tropical cyclones.
16.3.3. Life Cycle
At locations where all of the necessary conditions are met (including any one trigger), the incipient tropical cyclones usually progress through the following intensification stages: tropical disturbance, tropical depression, tropical storm, tropical cyclone.
16.3.3.1. Tropical Disturbance
The US National Hurricane Center defines a tropical disturbance as “a discrete tropical weather system of apparently organized convection (generally 200 to 600 km in diameter) originating in the tropics or subtropics, having a nonfrontal migratory character, and maintaining its identity for 24 hours or more.” Namely, it is a cluster of thunderstorms that stay together as they move across the ocean.
This thunderstorm cluster is visible by satellite as consisting of distinct thunderstorms with their own anvils and separate precipitation regions. There is no eye, and little or no rotation visible. Some tropical disturbances form out of Mesoscale Convective Systems (MCSs), particularly those that develop a Mesoscale Convective Vortex (MCV, see the Thunderstorm chapter). Some are identified as the thunderstorm clusters that move with easterly waves.
Most tropical disturbances do not evolve into tropical cyclones. Hence, tropical disturbances are not usually named or numbered. However, tropical meteorologists watch them carefully as they evolve, as potential future tropical cyclones.
As condensation and precipitation continue in this thunderstorm cluster, more and more latent heat is released in those storms. Namely, the mid-tropospheric air near this cluster becomes warmer than the surrounding ambient air. According to the hypsometric relationship, pressure decreases more slowly with increasing altitude in this warm region. Thus, synoptic-scale high pressure starts to form near the top of the troposphere in the region of this cluster.
16.3.3.2. Tropical Depression (TD)
The high pressure aloft begins to create a thermal circulation (see General Circulation chapter), where air aloft is driven horizontally outward — down the pressure gradient toward the lower pressure outside of the cluster. This diverging air aloft also starts to rotate anticyclonically.
The outward moving air aloft removes air molecules from the thunderstorm cluster region of the troposphere, thereby lowering the surface pressure under the cluster. The “depressed” pressure at sea level is why this stage gets its name: tropical depression. Tropical depressions are given an identification number, starting with number 1 each year in each region.
As this weak surface low forms, it creates a pressure gradient that starts to suck winds horizontally toward the center of the low. This inflowing air begins rotating cyclonically due to Coriolis force. The surface low pressure is usually too weak to measure directly. However, this tropical depression stage is defined by measurable near-surface winds of 17 m s–1 or less (Table 16-4) turning cyclonically as a closed circulation around the cluster. There is still no eye at this stage, however the thunderstorms are becoming aligned in spiral rain bands (Fig. 16.18). Most tropical depressions do not strengthen further.
Table 16-4. Stages leading to tropical cyclones. Mmax = near-surface wind-speed maximum. n/a = not applicable. | |||
Stage |
Mmax | ||
---|---|---|---|
(m s–1) | (km h–1) | (knots) | |
Tropical Disturbance | n/a | n/a | n/a |
Tropical Depression | < 17 | < 61 | ≤ 33 |
Tropical Storm | 17 - 32 | 61 - 118 | 33 - 63 |
Tropical Cycl.(Hurricane) | ≥ 33 | ≥ 119 | ≥ 64 |
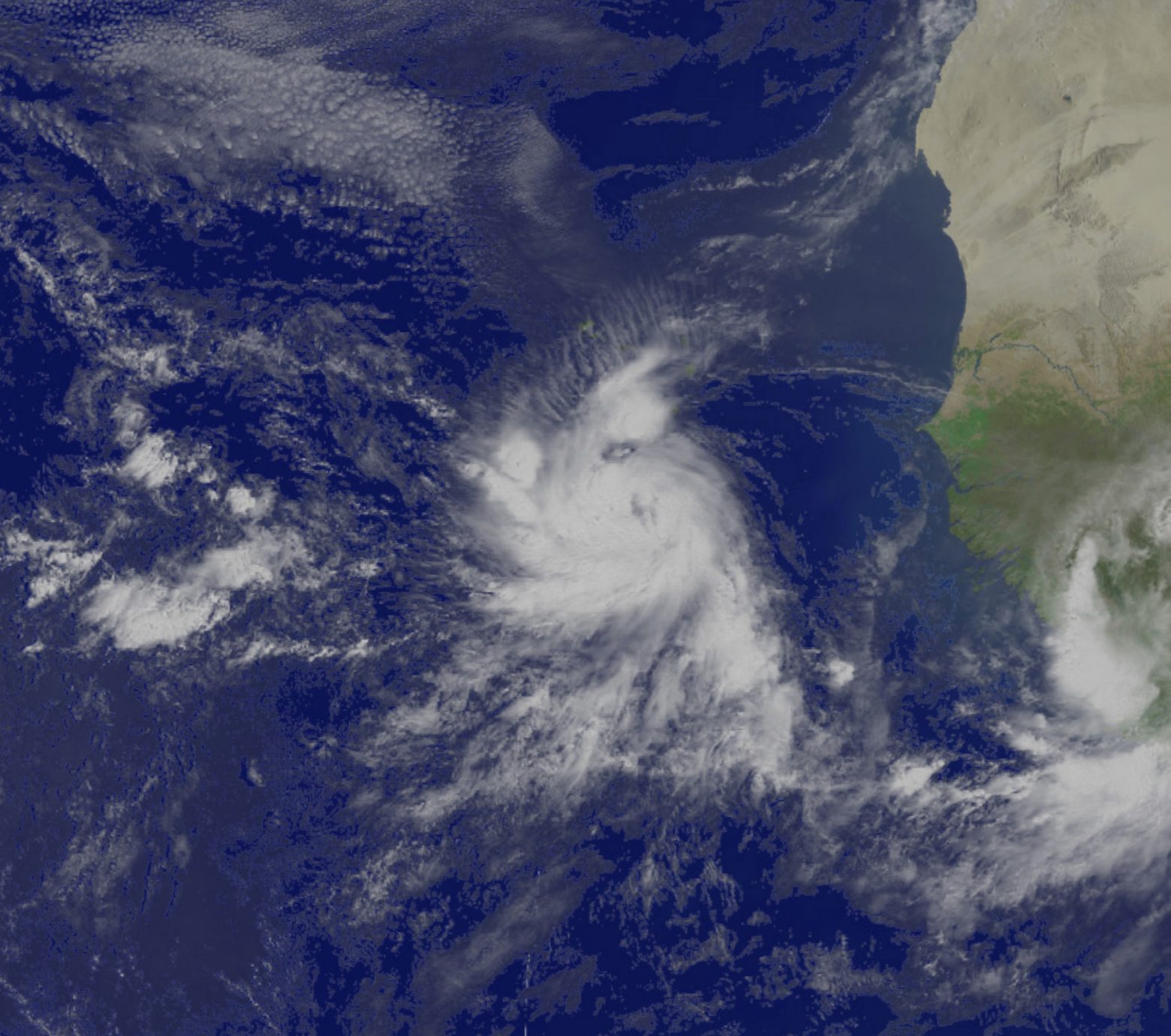
However, for the few storms that do continue to strengthen, the near-surface winds are boundarylayer gradient winds that spiral in towards the center of the cluster. This radial inflow draws in more warm humid boundary-layer air, thereby refueling the thunderstorms and allowing them to persist and strengthen. Condensation and precipitation increase, causing a corresponding increase in latentheat release.
This warm core of the storm further strengthens the high pressure aloft via the hypsometric relationship, which drives more outflow aloft and removes more air molecules from the troposphere near the cluster. Thus, the surface low pressure can continue to deepen in spite of the boundary-layer inflow. Namely, the high pressure aloft creates an exhaust system, while the boundary layer is the intake system to the incipient cyclone.
16.3.3.3. Tropical Storm (TS)
When the surface low is deep enough to drive winds faster than 17 m s–1 (but less than 33 m s–1) in a closed cyclone circulation, then the system is classified as a tropical storm. On weather maps, it is indicated with symbol
At this point, the thunderstorm rain bands have nearly completely wrapped into a circle — the future eyewall (Fig. 16.19d). The anvils from the thunderstorms have usually merged in to a somewhat circular central dense overcast (CDO), which is clearly visible as a large-diameter cold high cloud. There is no eye at this stage. Tropical storms are organized sufficiently to be able to modify their local environment to allow them to persist, without relying so much on a favorable ambient environment.
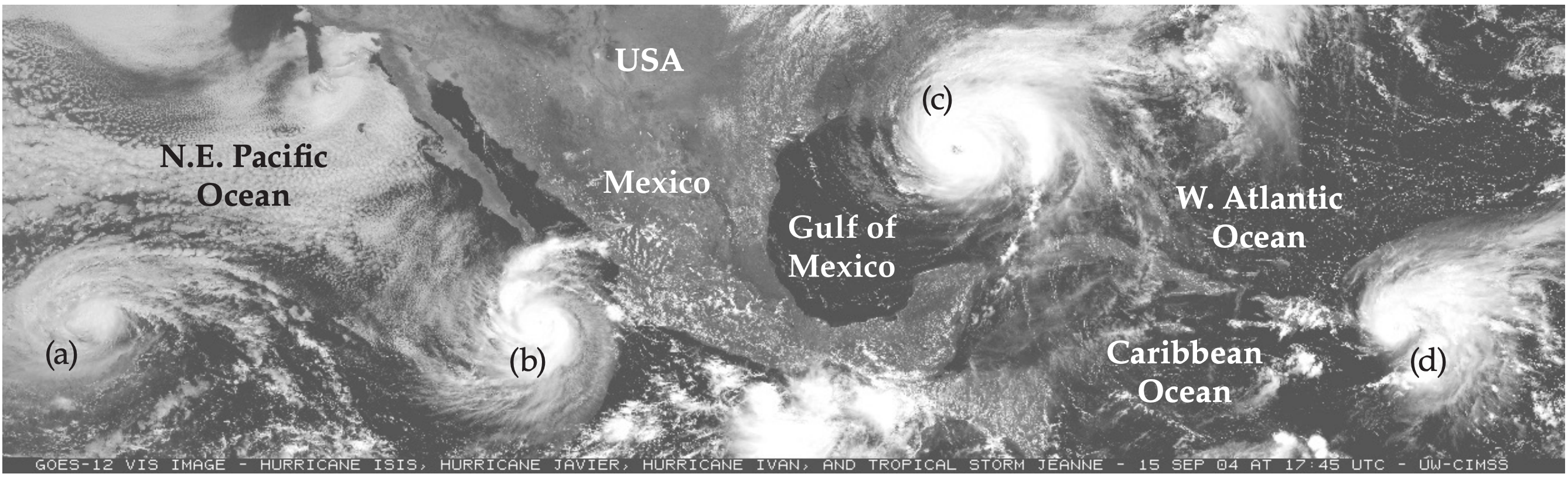
At this stage the storm is given a name for identification (and its former tropical-depression number is dropped). The same name is used if the storm further strengthens into a tropical cyclone, and for the rest of its life cycle.
Each ocean region has its own naming convention for these tropical storms. Hurricanes (Atlantic and Eastern Pacific) and Cyclones (Indian Ocean and Southwestern Pacific near Australia) are usually given names of men or women. These are assigned in alphabetical order according to lists that have been set in advance by the World Meteorological Organization (WMO). Typhoons are given names of flowers, animals, birds, trees, foods (not in alphabetical order but in an order set by the WMO’s Typhoon Committee).
Tropical-storm and tropical-cyclone names are re-used in a six-year cycle. However the names of the strongest, most destructive tropical cyclones are “retired” and never used again (they are replaced with other names starting with the same letter).
16.3.3.4. Tropical Cyclone (Hurricane, Typhoon)
Roughly half of the tropical storms in the Atlantic continue to strengthen into tropical cyclones. By this stage there is a well defined eye surrounded by an eyewall of thunderstorms. Max wind speeds are 33 m s–1 or greater in a closed circulation around the eye. The central dense overcast usually has a cloudfree eye. The weather map symbol for a tropical cyclone is .
There is heavy precipitation from the eyewall thunderstorms, and the core of the storm is significantly warmer than the environment outside the storm. Sea-level pressure decrease in the eye is measurable and significant, and a rise of sea level into a storm surge is possible. The storm organization allows it to persist for days to weeks as it moves across ocean basins (Fig. 16.19a-c).
16.3.4. Movement/Track
16.3.4.1. Steering by the General Circulation
Tropical cyclones are moved by the larger-scale winds near them. These winds are variable, causing a wide variety of hurricane tracks and translation speeds. However, by focusing on the climatological average trade winds and the monsoon circulations (Figs. 16.7 & 16.20), we can then anticipate average movement of tropical cyclones.
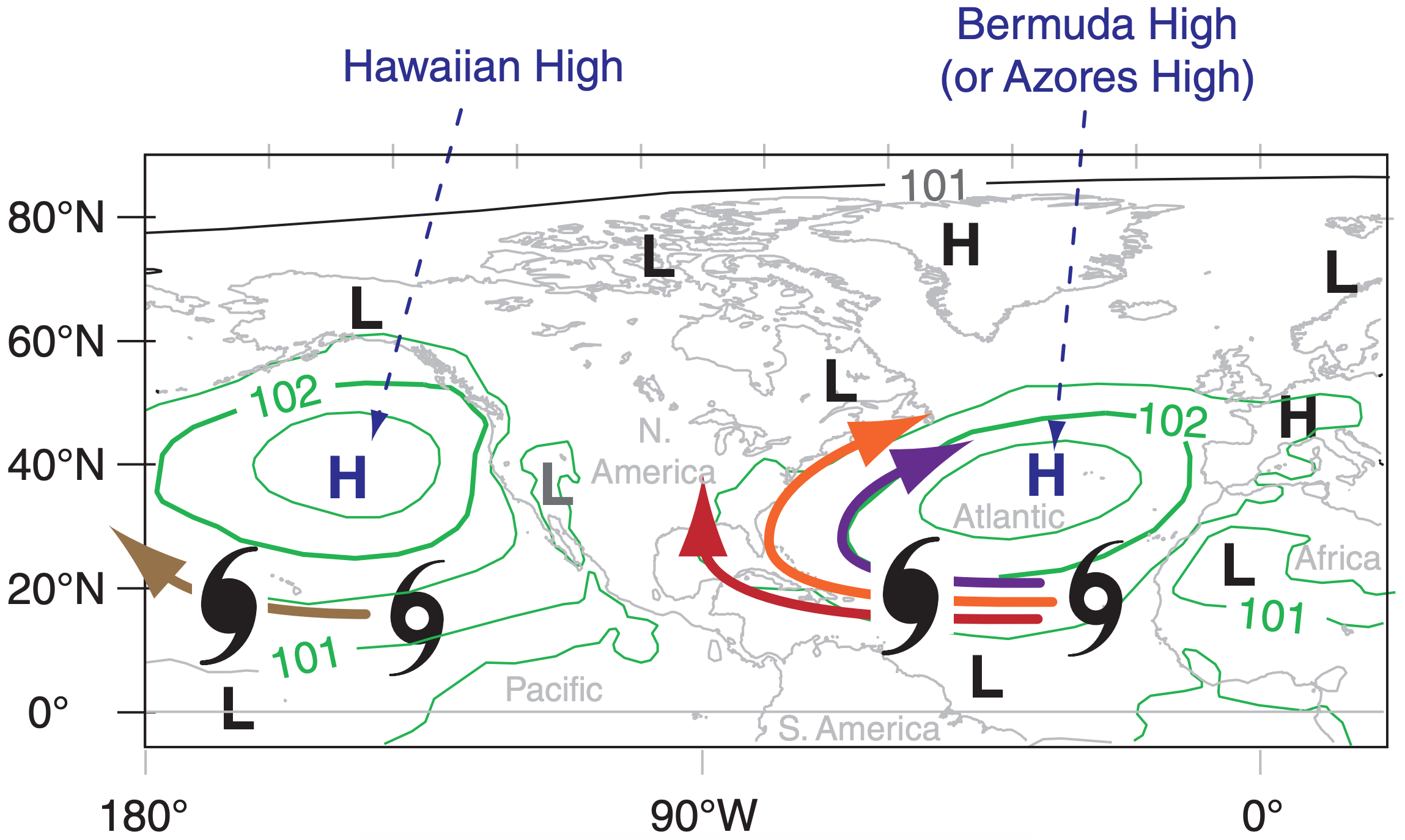
During late Summer and early Fall, monsoon high-pressure regions form over the oceans, as was discussed in the General Circulation chapter. Winds rotate clockwise (counterclockwise) around these highs in the Northern (Southern) Hemisphere. Equatorward of the high center the monsoon winds are in the same direction as the trade winds — from east to west. Thus, average tropical cyclone tracks in the eastern and central longitudes of an ocean basin are usually zonal — from east to west.
However, near the west sides of the ocean basins, the monsoon winds turn poleward (Fig. 16.20), and thus steer the tropical cyclones away from the equator. Many of these tropical cyclones travel along the east coast of continents in a poleward direction. The tracks vary from year to year, and vary within any one year (Fig. 16.20). Some of the tracks in the Atlantic might never hit N. America. Other tracks can be along the East Coast of North America, causing significant damage to coastal cities. Yet other tracks continue further westward across the Caribbean Sea and into the Gulf of Mexico before reaching the mainland.
16.3.4.2. Extratropical Transition
As the monsoon circulations move the tropical cyclones poleward, the cyclones leave the tropics and subtropics and enter the mid-latitudes. Here, prevailing winds are from the west in the global circulation. Also, winds on the poleward side of the monsoon highs are from the west. Thus, many tropical cyclone tracks eventually turn towards the east at midlatitudes.
Extratropical transition is the name given to the movement of tropical cyclones out of the tropics and into the midlatitudes. At these latitudes the storms are generally moving over colder water, causing them to rapidly die. Nonetheless, these former tropical cyclones can change into extratropical cyclones and still cause damaging floods, wind, and waves if over coastal areas. Even dying tropical cyclones well away from a coastline can influence midlatitude weather downwind by pumping copious amounts of moisture into the air. This humid air can later serve as the fuel for mid-latitude cyclones thousands of kilometers downwind, creating heavy rain and possible flooding.
16.3.5. Tropical Cyclolysis
Tropical cyclones can exist for weeks because they have the ability to create their own fuel supply of warm humid boundary-layer air. They create this fuel by tapping the heat and water stored in the upper ocean. Tropical cyclones die (tropical cyclolysis) when they move to a location where they cannot create their own fuel, or if they are destroyed by larger-scale weather systems, as described next.
16.3.5.1. Movement over Land
If the global circulation steers a tropical cyclone over land, it begins to die rapidly because it is unable to tap the warm ocean for more fuel (Fig. 16.21). If the land is a small island or narrow isthmus, then the weakened tropical cyclone can possibly re-intensify when it again moves over warm ocean water.
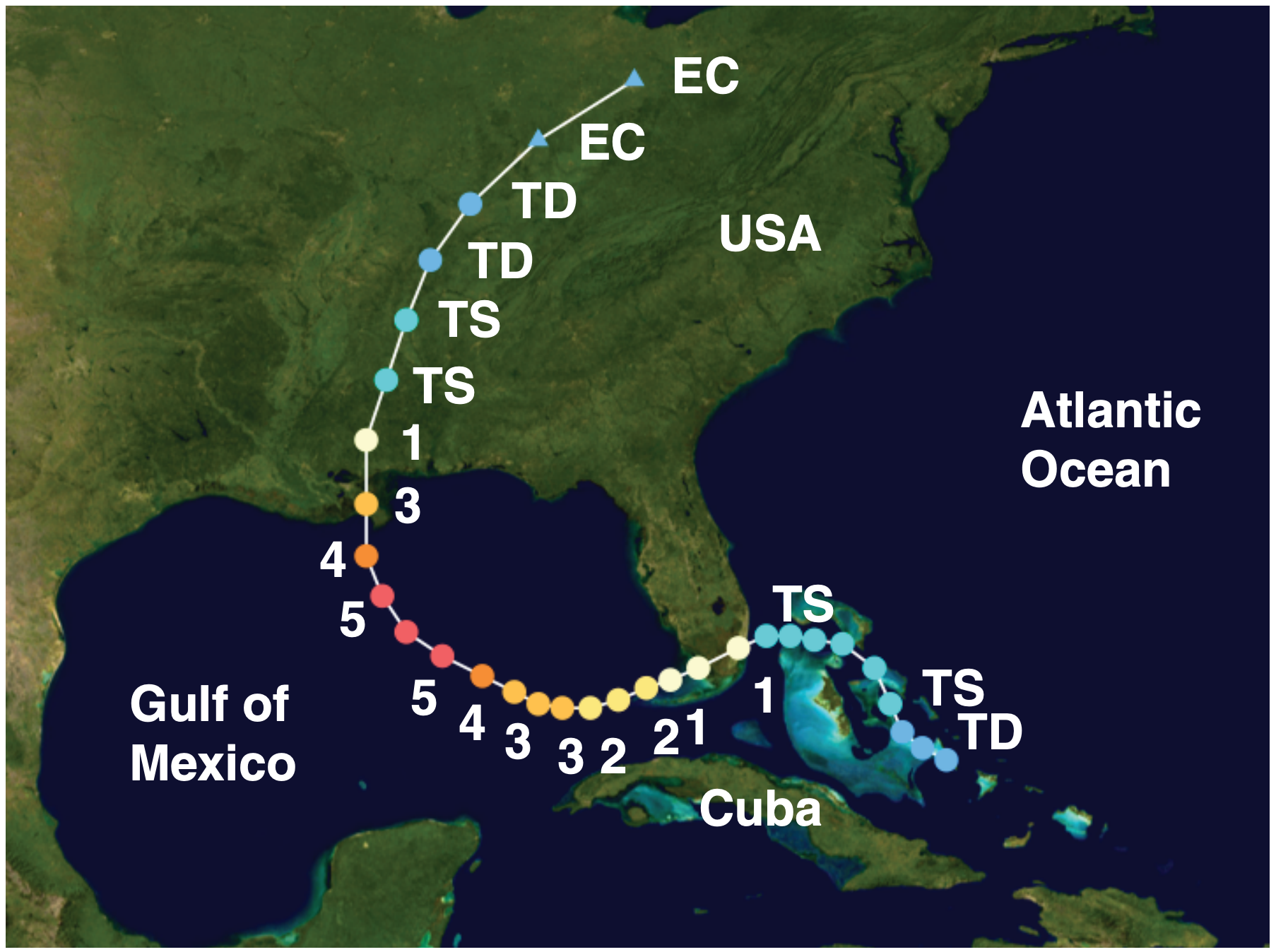
16.3.5.2. Movement over Cold Water
If the global circulation steers a tropical cyclone over water colder than 26.5°C, then it is unable to create new warm humid air as fast the eyewall thunderstorms consume this fuel. As a result, tropical cyclones quickly weaken over cold water.
This often happens when tropical cyclones are steered poleward toward mid-latitudes, where sea surface temperatures (SST) are colder (Fig. 16.8). In the Atlantic, the Gulf Stream of warm ocean water moving north along the east coast of the USA allows tropical cyclones there to survive. However, further north, the very cold Labrador Current (Fig. 16.22) moving south from the Arctic along the east coast of Canada causes most tropical cyclones to diminish below tropical cyclone force before making landfall (see INFO Box on next page).

Tropical cyclolysis can also occur if the layer of warm ocean water is too shallow, such that tropical cyclone-induced ocean waves stir colder deep water to the surface. Also, if a tropical cyclone crosses the path of a previous tropical cyclone, the ocean surface along this previous path is often colder due to the wave stirring.
16.3.5.3. Interaction with Mid-latitude Lows
When tropical cyclones move into mid-latitudes, they can encounter the larger, more powerful mid-latitude cyclones (Fig. 16.23) that were discussed in a previous chapter. Strong mid-latitude cold fronts can inject cold air into the tropical cyclone, causing the tropical cyclone to die. The fronts and associated jet stream aloft often have strong wind shear, and can rip apart the tropical cyclones. Sometimes tropical cyclones will move into the path of a mid-latitude cyclone, allowing the mid-latitude cyclone to capture some of the tropical cyclone’s energy and consume the tropical cyclone as the two systems merge.
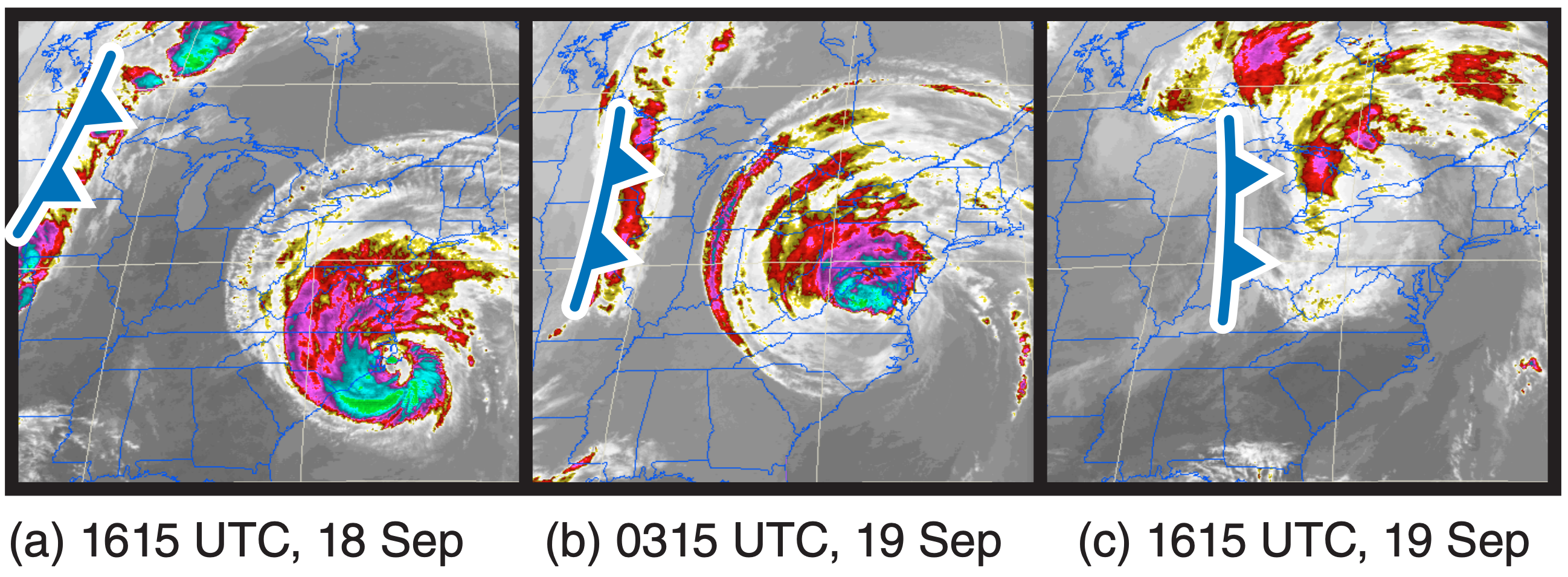