61.2: Sedimentary way-up structures
- Page ID
- 22870
We’ll begin with an examination of way-up structures in sedimentary deposits, whether lithified into sedimentary rock or in an unconsolidated state (but after deposition).
Cavity fills

When an empty space exists in a sedimentary deposit, such as the protected little hollow under a shell, it can be partially filled by sediment. We call this little pocket of space a “void” or a “cavity.” If the cavity is entirely filled with sediment, it’s useless as a geopetal structure. It only has geopetal value if it is partially filled in with mud. In fact, this is geopetal in the strictest sense of the term: it refers to these cavity fillings. Here is how it works: the mud settles under the influence of gravity, lining the bottom of the space (but not the top of the space). Later, as groundwater moves through the sediment, it carries with it dissolved ions, which may bond together, filling the available space with crystals. In the example at right, the crystals formed are of the mineral calcite, which occurs in a coarse form geologists call “spar.”
Use this principle to examine the following image, showing a cross-section through a bed of limestone collected in West Virginia. We have intentionally placed it in a vertical position for you to examine. As you will see, the limestone includes many snail shells (gastropod fossils). A lot of them show this key pattern of infilling with two substrates: gray limy mud and white calcite spar. Note that because the shells are made of calcite also, both the original shell and the subsequent spar appear the same coarse crystalline white:
Which way is depositional “up” in this sample? You can use the rotation tool at lower right to test various orientations, until you get the mud at the (original) bottom and the spar above it in the (original) top of the available space.
Hopefully you determined that the side of the sample next to the pencil was originally “down.”
The sample principle applies with minerals other than calcite. For instance, consider this slab of “Turitella agate,” a rock made of whole snail shells filled in with the tiny, clear, bean-shaped shells of small arthropods called ostracodes.
Compare and contrast these two shells from the slab, with their different way-up implications:


Explore the slab on your own if you want to search for additional examples. Or if you are ready to test yourself on a similar sample, from Texas, take the quiz below:
Did I Get It? - Quiz
Is this sample right-side-up or up-side-down? Explain.
a. Right-side-up, since the bottom is filled with mud, and the empty cavity at the top is partially filled with sparry calcite crystals.
b. Up-side-down, since the bottom is partially filled with sparry calcite crystals, and the top is filled with mud.
- Answer
-
a. Right-side-up, since the bottom is filled with mud, and the empty cavity at the top is partially filled with sparry calcite crystals.
Examine this sample for cavity fills.
Which way is depositional "up?"
a. The top of the screen (where the scale is) is the original depositional "up" direction.
b. The left of the screen (where the biggest shells are) is the original depositional "up" direction.
c. The bottom of the screen (opposite the side where the scale is) is the original depositional "up" direction.
- Answer
-
a. The top of the screen (where the scale is) is the original depositional "up" direction.
Crossbedding
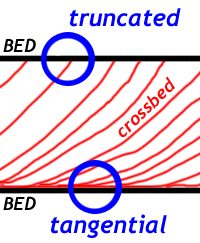
Cross-bedding is a series of laminations included within a larger sedimentary bed. A directional current allows the laminations to build up on the leeward (downstream) side of a migrating bedform called a ripple. (Bigger versions of ripples are called dunes, and they create cross-beds as they migrate, too.) Ripples and dunes form in a directional current of either water or air. They accumulate at the angle of repose for that size of sedimentary particle, and within that medium (saltwater, freshwater, or air). In general, it is a gentle angle of around 30\(^{\circ}\) of dip.
In terms of shape, cross bedding is most useful when the cross beds show a pronounced concavity, with the scoop-shape curving upward, like a smiley face. This concave-up shape is a reflection of the cross-beds curving into a gentler orientation, approaching parallel to the base of the main bed. We call this “tangential,” from the geometric term describing a line intersecting a circle at one point. At the top of the bed, in contrast, they are often truncated (“stopped short” or “cut off”), because the original upper tangential portion of the leeward side of the ripple has evidently been “planed off” by post-depositional erosion.
This leads to important insights, as shown in the case study of the Mixed-Up Quartzites of Cape Agulhas.
Examine this gigapixel panorama of a specimen of cross-bedded red sandstone. There are two halves to the sample shown. One is right-side-up. The other is up-side-down. Explore the two samples to see if you can figure out which one is which, applying the criteria explained above.
Really large cross-beds form via aeolian (wind) deposition in dune fields. The shape and geopetal implications are the same, but the cross-beds are much larger. Here is an example (right-way-up) from coastal exposures in the western Orkney Islands:
Exceptions to the rule
Cross-bedding can be tricky. Sometimes cross-bed laminae accumulate without the tangential, concave-up portion, and sometimes they preserve the upper convex-up portion at the ripple crest. Even crazier, sometimes the accumuate not on the leeward side of a dune, but the upstream (stoss) side! Let us take a moment to see what these complications look like...
CLIMBING RIPPLES
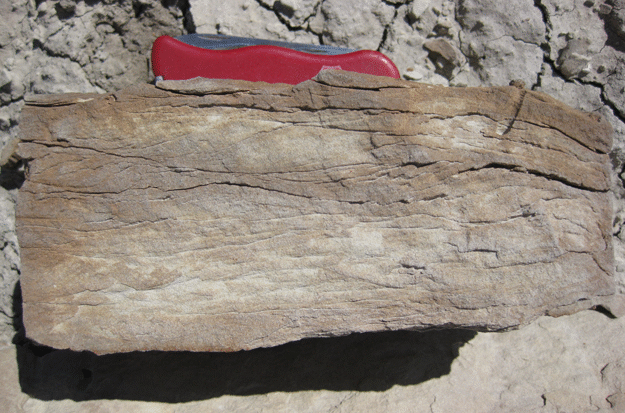
Climbing ripples form when downstream migration of a ripple or dune is accompanied by rapid vertical aggradation of sediment. This tends to occur when the sedimentary load is higher than the capacity of the current that’s carrying it. Climbing ripples are distinct structures indicating critical to supercritical flow, but they often look about the same right-side-up as they do up-side-down. The concave-up portion of the laminae in the lee of the ripple is matched by the convex-up crest of the ripple itself, buried as soon as it forms. As a result, they can mislead the historical geologist.
Consider the example below; if you flipped the photo up-side-down, it would be difficult to tell:


The image at right (click to enlarge) shows a cross-section through three sets of climbing ripples from modern (unlithified) sand deposits in the Mississippi Delta region of Louisiana. Note how the largest, oldest, deepest set of cross beds shows an undulating set of internal laminations, and these “climb” up and to the right, with tangential bases. This is likely indicative of supercritical flow. The second set has a trough-like bottom that cuts into the older set of cross-beds, and is truncated by the most recent, uppermost, thinnest set of cross-beds.
Finally, here is a gigapixel panorama of a specimen showing climbing ripples in cross-section:
ANTIDUNES

If water is flowing fast enough to be in the upper flow regime, antidunes can form. They are mostly found in shallow channels (e.g., fluvial and tidal channels). If you are lucky enough to see them forming, you can recognize the situation when you see standing surface waves – watch closely and you will see the waves migrate upstream over time, meaning that the are bedforms developing immediately below the standing waves. If high flow is maintained, the antidunes will also migrate upstream. They do this by adding new sediment on their upstream (stoss) side rather than the usual situation for migrating ripples, which deposit sediment on the downstream (lee) side. So antidune cross-beds dip in the opposite direction from normal ripple or dune cross-beds. The good news is that the preservation potential of antidunes is low, because as soon as flow slackens they tend to wash out and be eroded.
Did I Get It? - Quiz
Is this outcrop of sandstone right-side-up or tectonically inverted? Why?
a. It must be tectonically inverted, since the cross-beds are up-side-down: they are truncated at the bottom, and tangential at the top.
b. It appears to be right-side-up, since the cross-beds in the middle of the outcrop show tangential relationships to the main bed at the bed's bottom, and are truncated at the top.
- Answer
-
b. It appears to be right-side-up, since the cross-beds in the middle of the outcrop show tangential relationships to the main bed at the bed's bottom, and are truncated at the top.
Is this sample of sandstone in its upright depositional position, or up-side-down?
a. It is right-side-up.
b. It is up-side-down.
- Answer
-
b. It is up-side-down.
Are these cross-beds likely to be fluvial (river-deposited) or aeolian (wind-deposited)? Why?
a. Fluvial, because the outcrop is broken by a couple of weathered-out joints (vertical fractures).
b. Aeolian, since they are so large.
c. Aeolian, because the outcrop has some snow on it.
d. Fluvial, since they are right-side-up.
- Answer
-
b. Aeolian, since they are so large.
Which way-up structure is shown here? Are the strata right-side-up, or up-side-down?
a. Graded bedding; the strata are up-side-down.
b. Cavity fills; the strata are up-side-down.
c. Climbing ripples; the strata are right-side-up.
d. Climbing ripples; the strata are up-side-down.
e. Cavity fills; the strata are right-side-up.
f. Graded bedding; the strata are right-side-up.
- Answer
-
c. Climbing ripples; the strata are right-side-up.
Which way is paleo-"up" in this sample of quartzite and red argillite? Why?
a. Paleo-"up" is to the right, on the opposite side from where the scale bar is. This is because the central set of cross beds are tangential to the main bed at the right, but truncated by white sand at the left.
b. Paleo-"up" is to the left, where the scale bar is. This is because the central set of cross beds are tangential to the main bed at the right, but truncated by white sand at the left.
- Answer
-
b. Paleo-"up" is to the left, where the scale bar is. This is because the central set of cross beds are tangential to the main bed at the right, but truncated by white sand at the left.
Ripple marks
Ripple marks are the 3D expression of the same phenomenon as cross-bedding: the actual bedform’s shape exposed in lithified form. They can be either symmetrical or asymmetrical. The 3D model below shows a great example of aysmmetric ripple marks, the kind that form in a unidirectional current. Rotate the model so you are looking down the crest of the central ripples so you can see their shape in cross-section: the left side is gently sloped compared to the right side. The left side is therefore the upstream (stoss) side, and the right side is therefore the downstream (leeward) side.
Here is a 3D model showing some ripple marks exposed on multiple bedding planes within the shallow-water Foreknobs Formation (Devonian):
One bedding surface shows fairly linear ridge crests, but an older (deeper) layer to the right shows interference ripples between two perpendicular wave orientations. These create little “point” like features that protrude outward from the bed in the paleo-“up” direction.
Did I Get It? - Quiz
What primary sedimentary structure is shown in this 3D model?
Which way was the current flowing when this sediment was deposited?
a. Ripple marks. Current flowed from location "5" toward location "3."
b. Ripple marks. Current flowed from location "3" toward location "5."
c. Ripple marks. Current flowed from location "4" toward location "2."
d. Ripple marks. Current flowed from location "2" toward location "4."
- Answer
-
b. Ripple marks. Current flowed from location "3" toward location "5."
Does this diagram show symmetrical or asymmetrical ripples?
a. Symmetrical
b. Asymmetrical
- Answer
-
b. Asymmetrical
Mud cracks
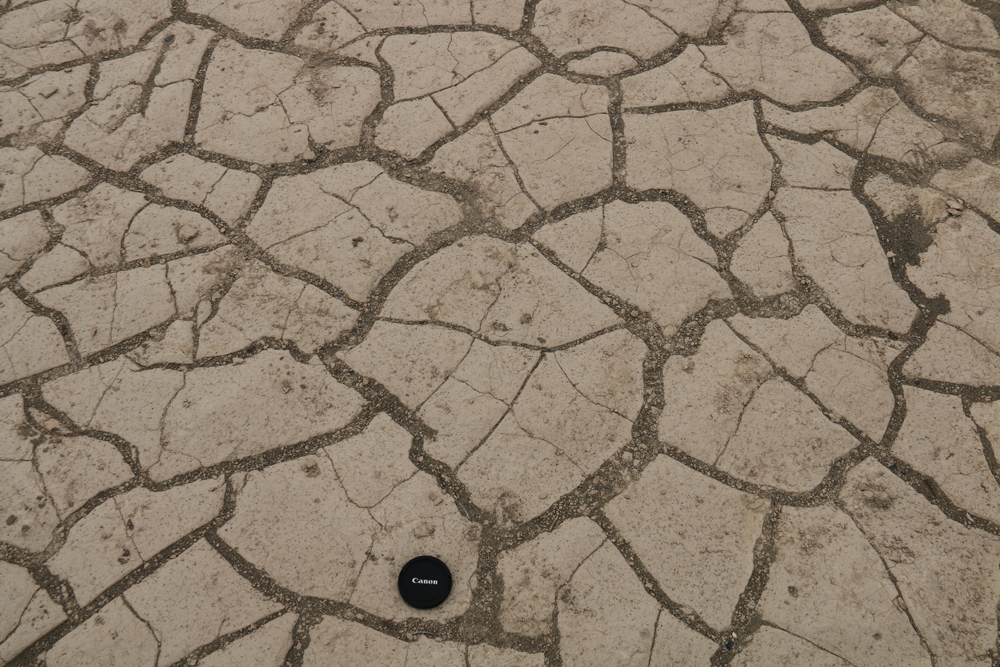
The desiccation of mud produces shrinkage when stresses induced by volume loss through evaporation are greater than the cohesive strength of the mud. Cracks initiate and grow, dividing the mud up into a series of irregular polygons. The photograph here shows the sort of thing we think about when we think of mudcracks: a vast dry field of polygons, sometimes with air between them, and sometimes filled with later sediment.
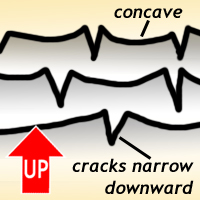
But the geopetal value of mud cracks comes from their cross-sectional view, which is perhaps not as intuitive but is worth considering, because it is very useful. The cracks propagate downward through the mud deposit in an ever-widening gap. This gap is widest at the top and most narrow at its deepest propagating tip, giving it an overall V shape in cross-section. When a deposit of new sediment gets dumped atop this open gap, it can fill it in. In cross-section, this creates a V-shaped projection down through the desiccated muddy layer.
This 3D model shows these aspects of the shape of well-developed desiccation cracks well:
In this gigapixel panorama, you can see a cross-sectional view of some red shale (former mud) and white sandstone (former sand), from the Triassic of Scotland. Note how the mud cracks appear in cross-section: almost like spiky white teeth in a red mouth!
Did I Get It? - Quiz
What primary sedimentary structure is shown here?
a. Cavity fill
b. Mud cracks (desiccation cracks)
c. Flame structure
d. Cross-bedding
e. Graded bedding
- Answer
-
b. Mud cracks (desiccation cracks)
What primary sedimentary structure is shown here?
a. Climbing ripples
b. Ball & pillow
c. Mud cracks (desiccation cracks)
d. Graded bedding
e. Cross-bedding
- Answer
-
c. Mud cracks (desiccation cracks)
Identify the primary sedimentary structure shown here. Are we looking at the top or the bottom of the bed? Explain.
a. Cross-bedding is shown. The current must have been flowing from right to left, and paleo-"up" is toward the bottom of the photo.
b. Cross-bedding is shown. The current must have been flowing from left to right, and paleo-"up" is toward the top of the photo.
c. Mud cracks are shown. Because the sandstone filling in the gaps between the mud cracks pokes outward from the face of the slab, this must be the underside of a sandstone bed that filled in the mudcracks. We are looking "upward" at the bottom of this post-mud-crack sand bed.
d. Mud cracks are shown. Because the sandstone filling in the gaps between the mud cracks pokes downward into the face of the slab, this must be the upper surface of the mud layer that dried out. We are looking "downward" at the top of this muddy layer.
- Answer
-
c. Mud cracks are shown. Because the sandstone filling in the gaps between the mud cracks pokes outward from the face of the slab, this must be the underside of a sandstone bed that filled in the mudcracks. We are looking "upward" at the bottom of this post-mud-crack sand bed.
What primary sedimentary structure is shown here? What are the implications?
a. Mud cracks: So mud must have been deposited by water, then dried out in the open air.
b. Graded bedding: sand and mud were deposited by a turbidity current, probably in a deep ocean setting.
c. Cross-bedding: sand was deposited by a flowing directional current of water, running from left to right.
- Answer
-
a. Mud cracks: So mud must have been deposited by water, then dried out in the open air.
What primary sedimentary structure(s) is/are shown? What are the implications?
a. Mud cracks are shown, as well as raindrop impressions and what appear to be automobile tire tracks. The sequence of events implied is (1) a vehicle driving over the mus, (2) a spattering of rain drops, (3) drying of mud, making mud cracks, and finally (4) deposition of mud.
b. Mud cracks are shown, as well as raindrop impressions and what appear to be automobile tire tracks. The sequence of events implied is (1) deposition of mud, (2) drying of mud, making mud cracks, (3) a vehicle driving over the mudcracks, and finally (4) a spattering of rain drops.
- Answer
-
b. Mud cracks are shown, as well as raindrop impressions and what appear to be automobile tire tracks. The sequence of events implied is (1) deposition of mud, (2) drying of mud, making mud cracks, (3) a vehicle driving over the mudcracks, and finally (4) a spattering of rain drops.
Graded bedding
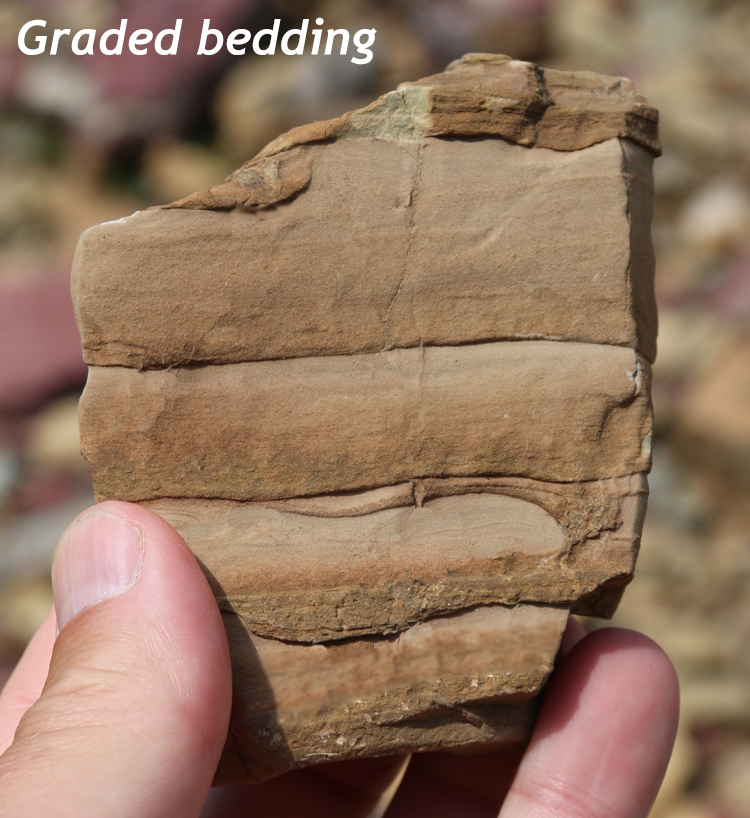
Graded bedding is a distinctive and widespread primary sedimentary structure where the grain size changes systematically from the bottom to the top of a bed. Because graded bedding forms as suspended grains settle from a turbidity current, the heaviest grains tend to settle out first, and those tend to be the biggest grains. A graded bed therefore has the largest grains at the bottom, and then they gradually get finer toward the top of the bed. This change in grain size within the bed is transitional and gradational, but the contact between graded beds will typically be quite crisp – a sudden switch from the fine grains at the top of one graded bed and coarser grains at the bottom of the overlying bed.
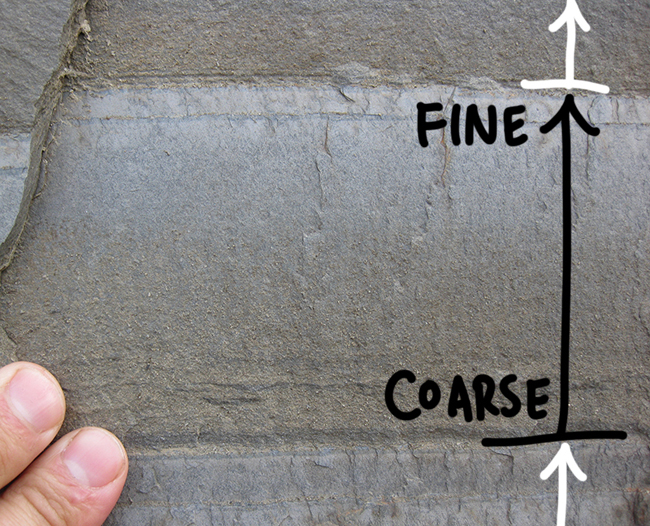
Graded bedding is most commonly found in submarine turbidity current deposits (e.g., on an abyssal fan), but it can occur in other settings too, such as lakes, floodplains, and tidal flats.
It also is not limited to siliciclastic rocks, and may be found in carbonate sedimentary deposits as well. In the GIGAmacro image below, you can examine an exquisite sample from the Silurian-aged tidal flat carbonates of the Tonoloway Formation (West Virginia), showing a series of graded beds and corroborating sedimentary structures (annotated in yellow circles) with ambiguous evidence (annotated with pink circles) and misleading evidence (annotated with the lone gray circle). Please click on the various annotations to learn the lessons this sample has to teach about interpreting which way is “up.”
Did I Get It? - Quiz
Examine this piece of limestone. Which way is "up?"
a. "Up" is up (in other words, where the scale bar is at the top of the screen is the original depositional "up" direction).
b. "Down" is up (in other words, the bottom of the screen, opposite the scale bar, is the original depositional "up" direction).
c. "Left" is up (in other words, counterclockwise from the scale bar, along the left edge of the screen, is the original depositional "up" direction).
- Answer
-
a. "Up" is up (in other words, where the scale bar is at the top of the screen is the original depositional "up" direction).
Examine this outcrop. What way-up structure is shown? Which way is up?
a. Flame structures are shown. The top of the photograph is the paleo-"up" direction.
b. Flame structures are shown. The bottom of the photograph is the paleo-"up" direction.
c. Graded bedding is shown. The top of the photograph is the paleo-"up" direction.
d. Graded bedding is shown. The bottom of the photograph is the paleo-"up" direction.
- Answer
-
c. Graded bedding is shown. The top of the photograph is the paleo-"up" direction.
Examine this outcrop. Which way is up?
(Note that there are some offsets of the bedding along small faults; don't let that throw you off.)
a. "Down" is up. The youngest part of this deposit is at the bottom, on the opposite side from where the pencil is.
b. "Up" is up. The youngest part of this deposit is at the top where the pencil is.
c. "Right" is up. The youngest part of this deposit is on the right side of the photo, underneath the shady overhanging chunk of rock.
- Answer
-
a. "Down" is up. The youngest part of this deposit is at the bottom, on the opposite side from where the pencil is.
Examine this outcrop. Which way is up?
a. The graded bed that starts in the middle of the outcrop gets coarser going downward, so these strata are right-side-up.
b. The graded bed that starts in the middle of the outcrop gets finer going downward, so these strata are up-side-down.
- Answer
-
b. The graded bed that starts in the middle of the outcrop gets finer going downward, so these strata are up-side-down.
Exceptions to the rule
In normal graded bedding, we see coarse at the bottom and fine at the top, with a gradational transition in between. Therefore, in most cases, we can find graded bedding in an outcrop of sedimentary rock or volcanic ash, and find the coarsest bit and head in the direction of the finest bit, and be confident the strata get younger in that direction. However, caveat emptor: nature is vast and varied, and she has a few more tricks up her sleeve.
REVERSE GRADING

For instance, there are natural examples of what is called “reverse graded bedding,” with exactly the opposite pattern. (It’s also called “inverse graded bedding.”) In particular, debris flows sort grains in the direction that to normal graded bedding would be considered “up-side-down.” In the example at left, we see an example from coastal California: an upright submarine debris flow recorded by a stack of muddy sand transitioning gradationally upward into coarse sand and pebbles, and finally to a surface scum of subangular cobbles. The biggest particles rise to the top due to a phenomenon called “the Brazil Nut Effect.”

RECRYSTALLIZATION
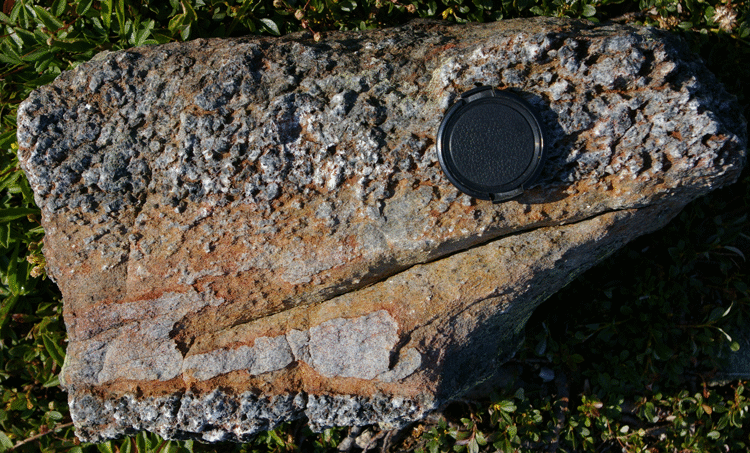
Not only that, but metamorphism can make it even more complicated. Consider this example from the Littleton Formation, in the Presidential Range of New Hampshire:
It shows a Devonian-aged turbidite that has undergone serious metamorphism. But not all the minerals in the rock reacted the same way to metamorphic conditions. The clay in the finer, upper part of the graded bed was more susceptible to metamorphic reactions than the quartz making up the sand in the coarser, lower part of the graded bed. As a result, not much happened with the quartz at high temperature and pressure, while the clay reacted profusely to generate huge porphyroblasts of andalusite — crystals many times larger than the grains of sand which were originally the coarsest components of the rock. So the sense of grading was reversed through metamorphic reactions. The key thing to recognizing this phenomena is to understand that the fine portion of a siliciclastic graded bed is likely made of different minerals (clay) than the coarse portion (quartz and perhaps feldspar) and so will show a different susceptibility to metamorphism under high pressure / temperature conditions.
This isn’t a fatal flaw, since we understand the metamorphic reactions that convert clay into metamorphic minerals such as garnet, staurolite, sillimanite, kyanite, and andalusite, and we know that quartz is chemically stable over a wide range of temperature and pressure conditions. In fact, we could consider this a blessing in disguise – because we get information both about the metamorphic conditions the rock experienced (andalusite only forms at low pressures and moderate temperatures) but we also still preserve the crisp/gradational/crisp character of the original graded beds. The primary sedimentary structure is altered, but not beyond recognition. It is not destroyed.
Did I Get It? - Quiz
What variety of graded bedding is shown in this upright layer of Absaroka volcanic breccia?
a. Recrystallized (metamorphically inverse-coarsened) graded bedding
b. Normal graded bedding
c. Reverse graded bedding
- Answer
-
c. Reverse graded bedding
Here is a normally graded turbidite sequence. Which way is paleo-"up?"
a. Down is paleo-"up," since the central sand body has a crisp edge near the black shale at the top, and the sand gets finer toward the bottom.
b. Up is paleo-"up," since the central sand body has a crisp edge near the black shale at the top, and the sand gets finer toward the bottom.
c. Left is paleo-"up," since the central sand body has a crisp edge near the black shale at the top, and the sand gets finer toward the bottom.
- Answer
-
a. Down is paleo-"up," since the central sand body has a crisp edge near the black shale at the top, and the sand gets finer toward the bottom.
Examine this sample. Which way is the paleo-"up" direction, and why?
a. Up is paleo-"up" because the beds are upright.
b. Right is paleo-"up" because the is a lot of garnet there, indicating the rich former mud at top of the graded bed.
c. Left is paleo-"up" because the beds show a gradation from quartz rich former sand at the base of a graded bed to garnet rich former mud at top of the graded bed.
- Answer
-
c. Left is paleo-"up" because the beds show a gradation from quartz rich former sand at the base of a graded bed to garnet rich former mud at top of the graded bed.
Does this outcrop show normal grading or reverse grading?
a. Normal grading; this looks like a turbidity current deposit.
b. Reverse grading; it must be a debris flow deposit.
- Answer
-
b. Reverse grading; it must be a debris flow deposit.
This outcrop is on the slopes of a volcano in Ecuador. Examine the layer behind the geologist's jacket. Identify the way-up structure there and interpret it.
a. Normal graded bedding. This is a submarine turbidity current deposit.
b. Reverse graded bedding. This is a lahar (volcanic mudflow) deposit.
- Answer
-
b. Reverse graded bedding. This is a lahar (volcanic mudflow) deposit.
Recap graded beds as way-up indicators with this slideshow:

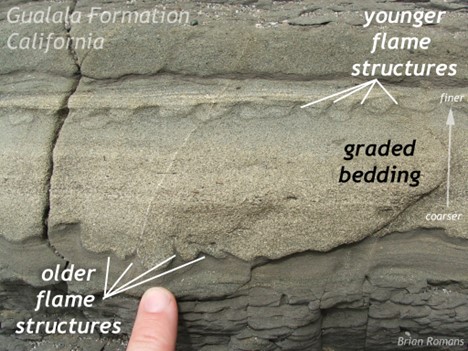
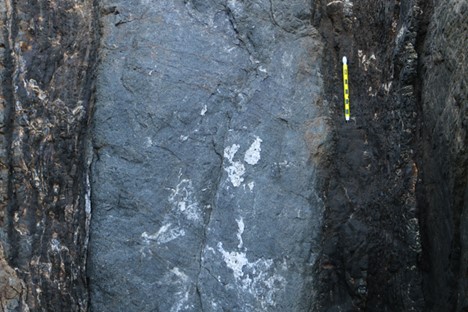
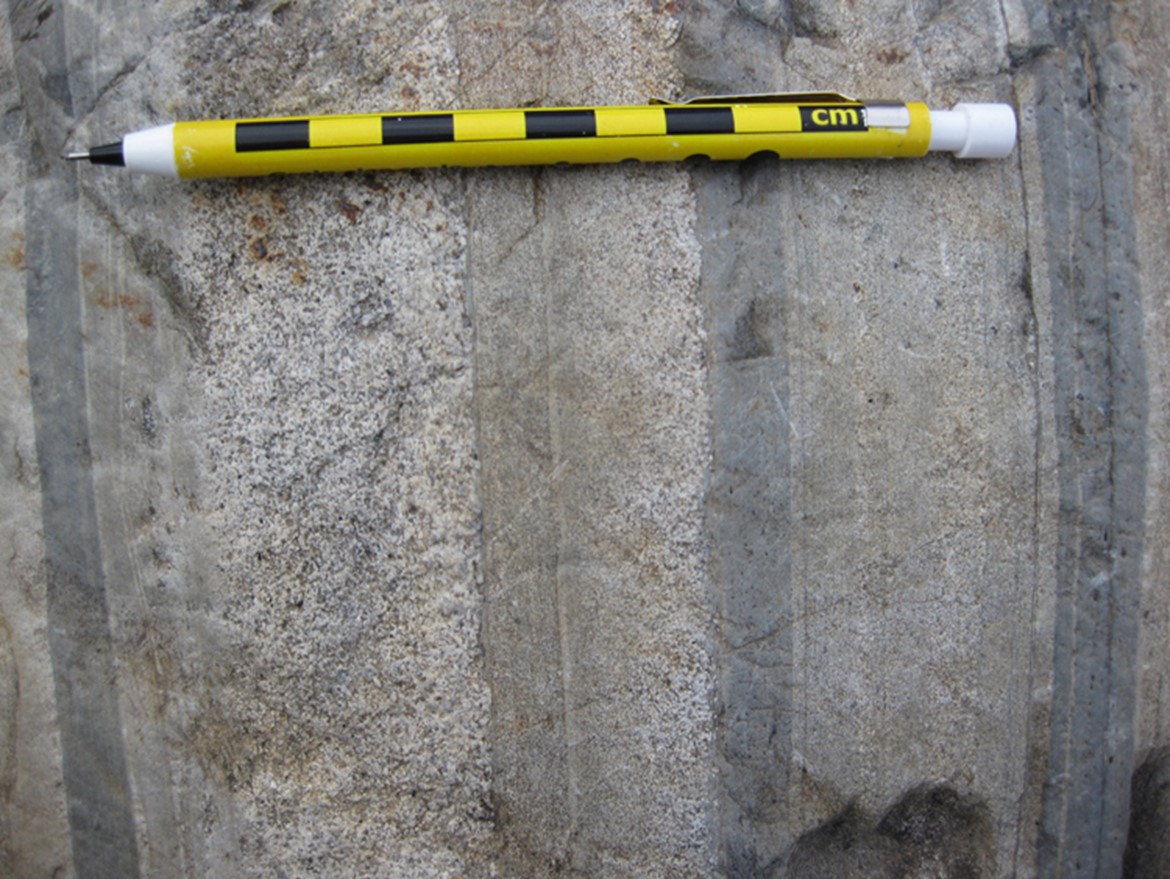
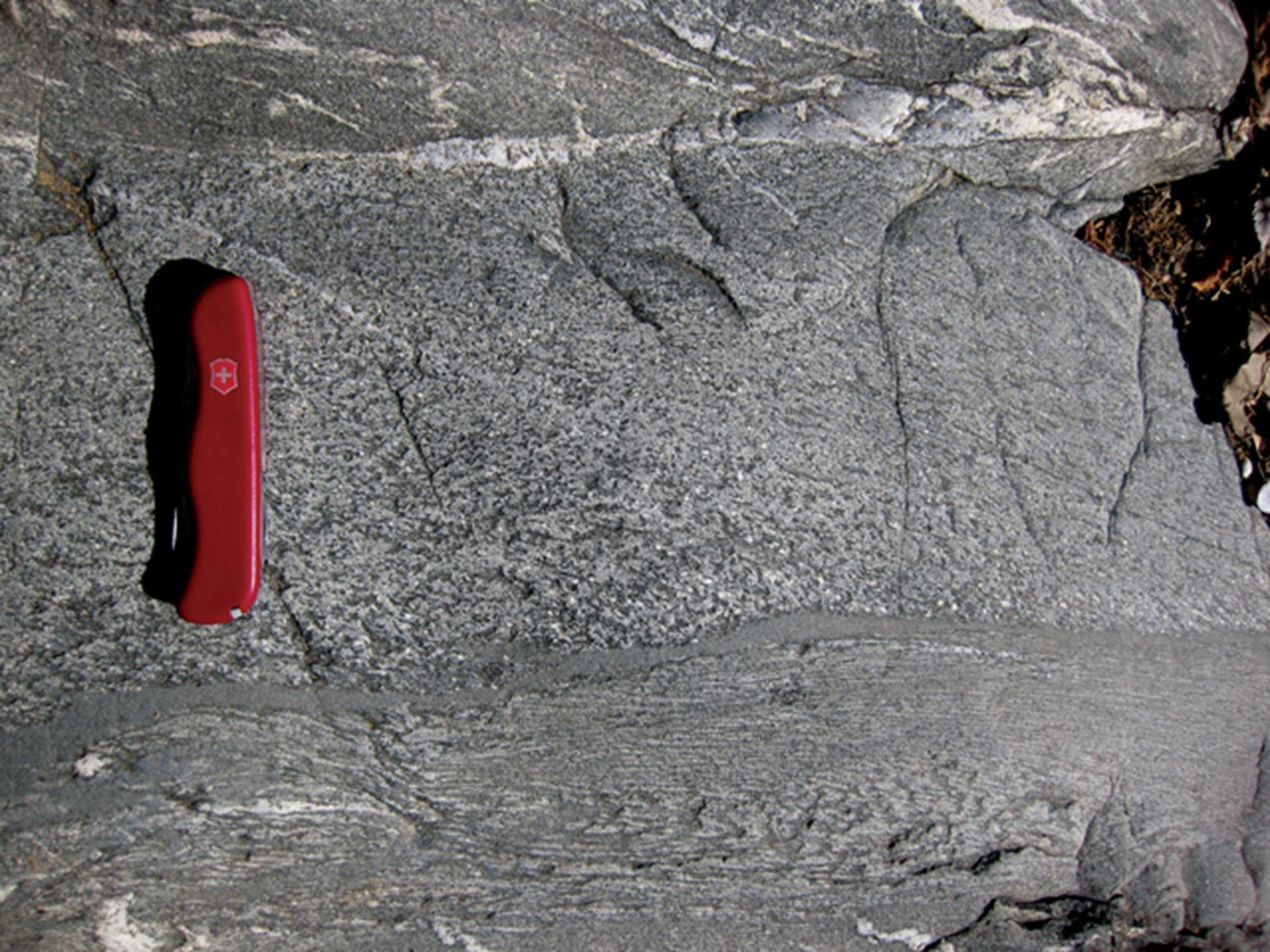

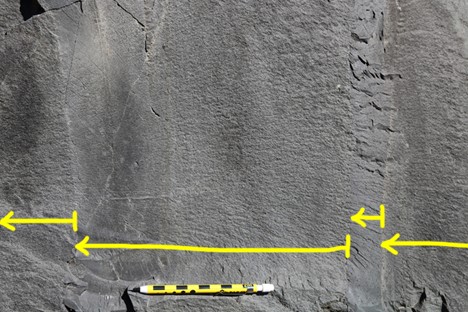
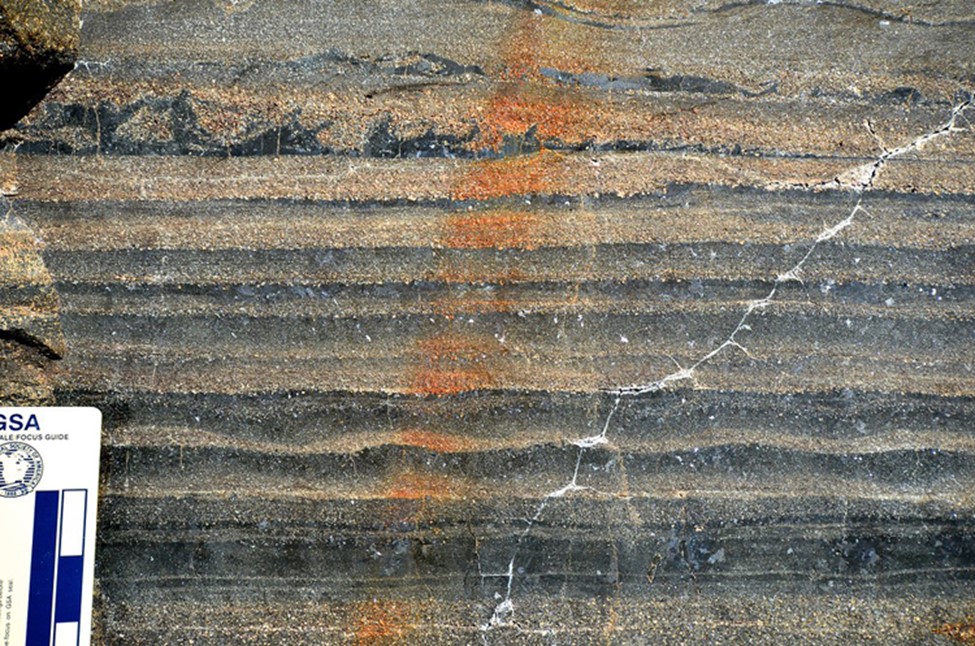
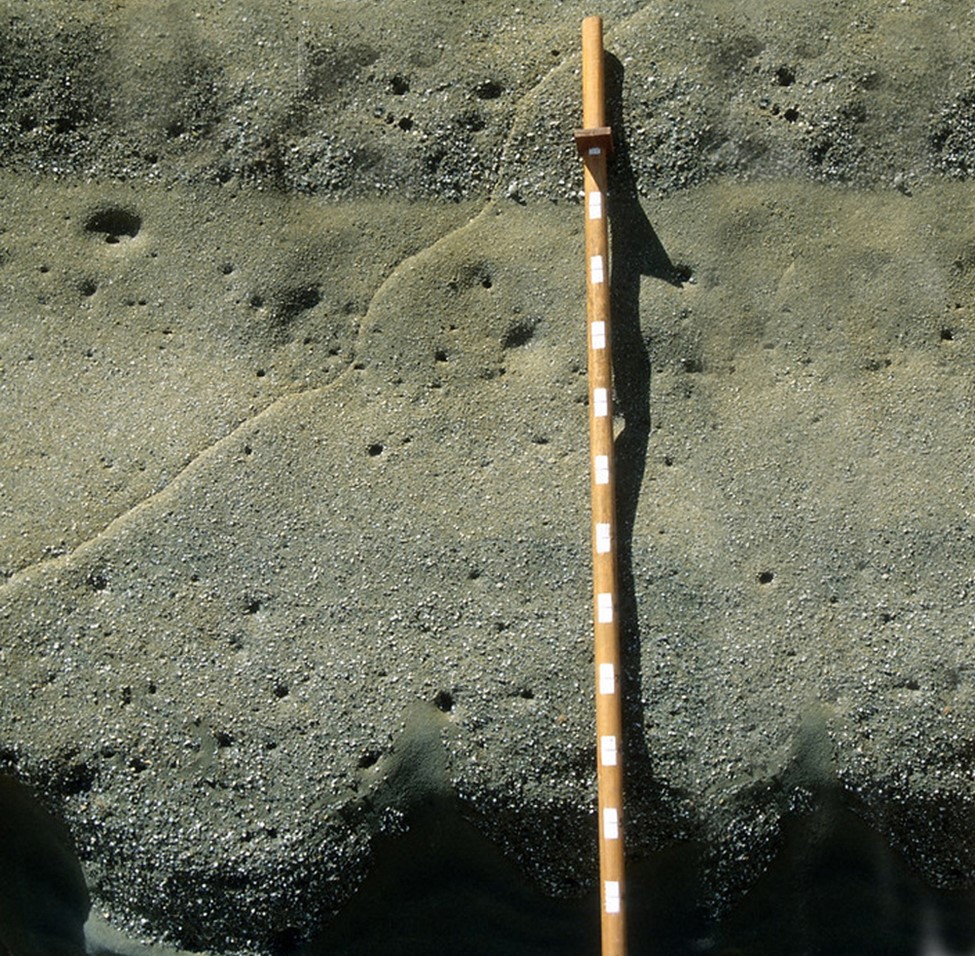

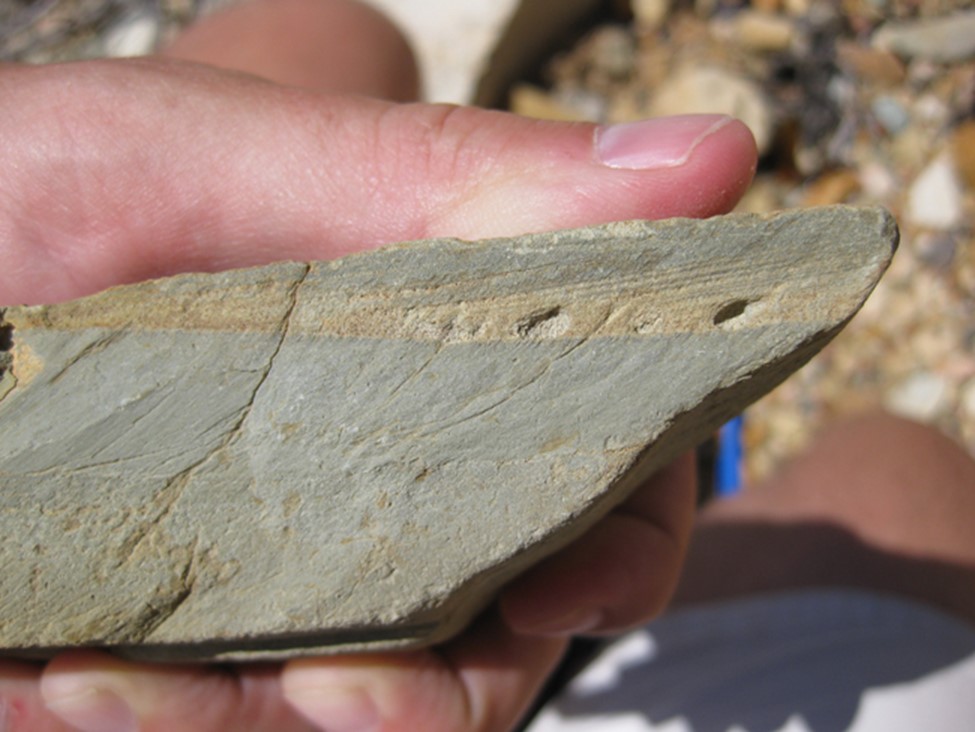
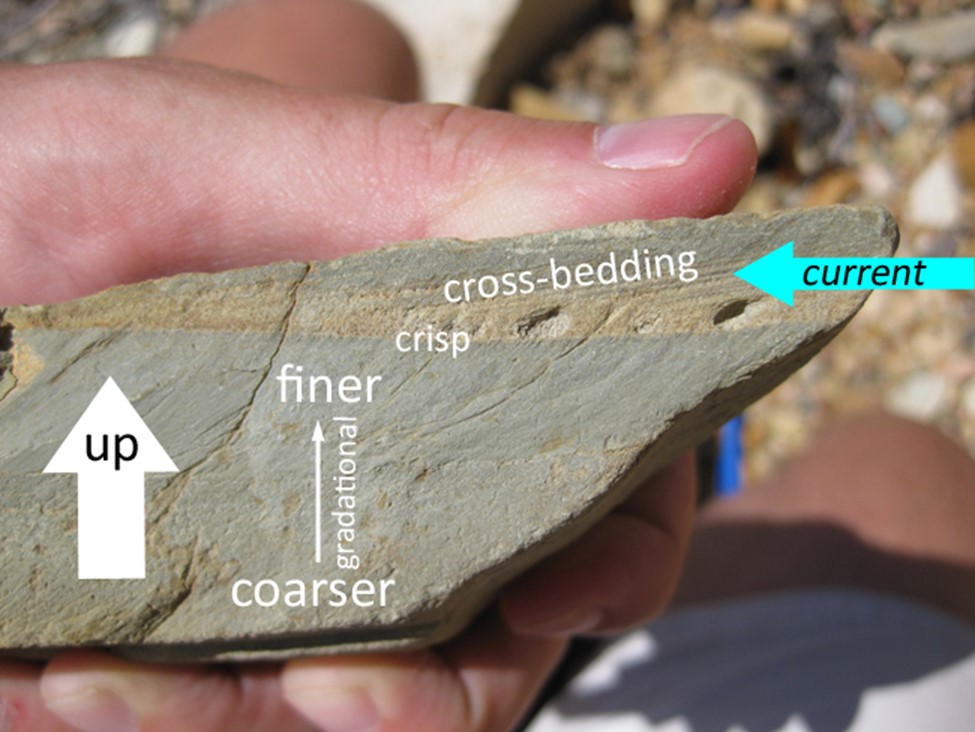

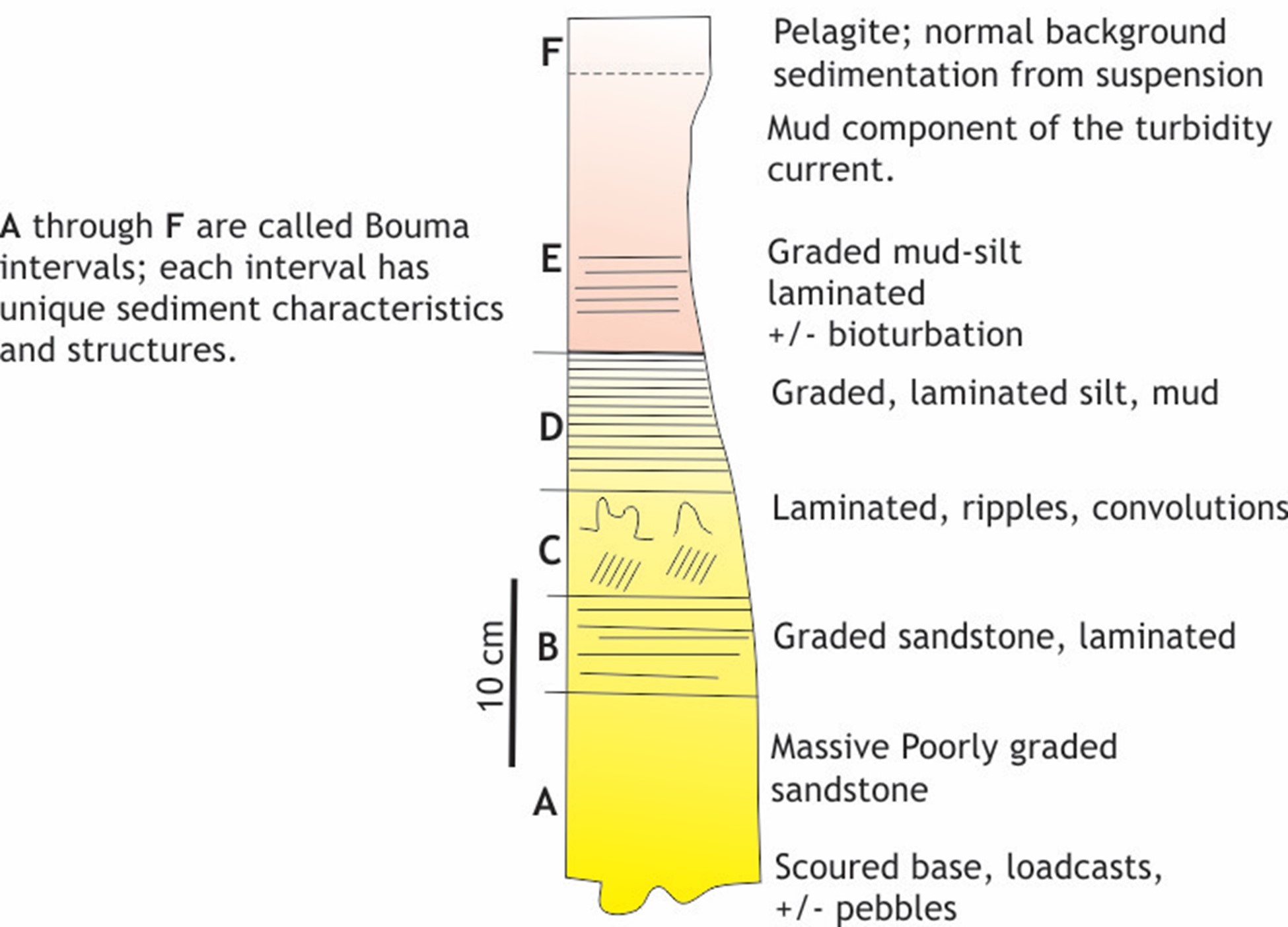

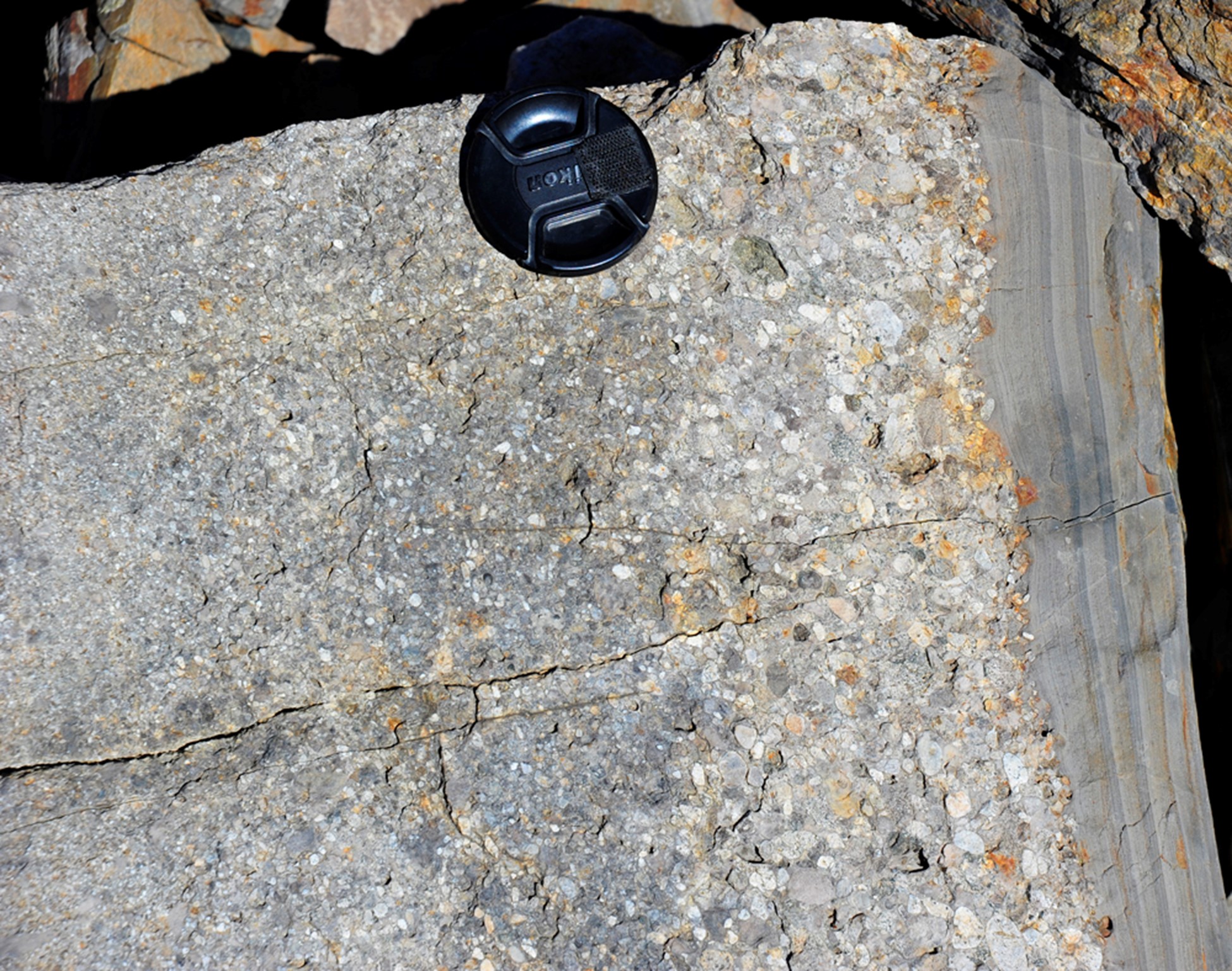
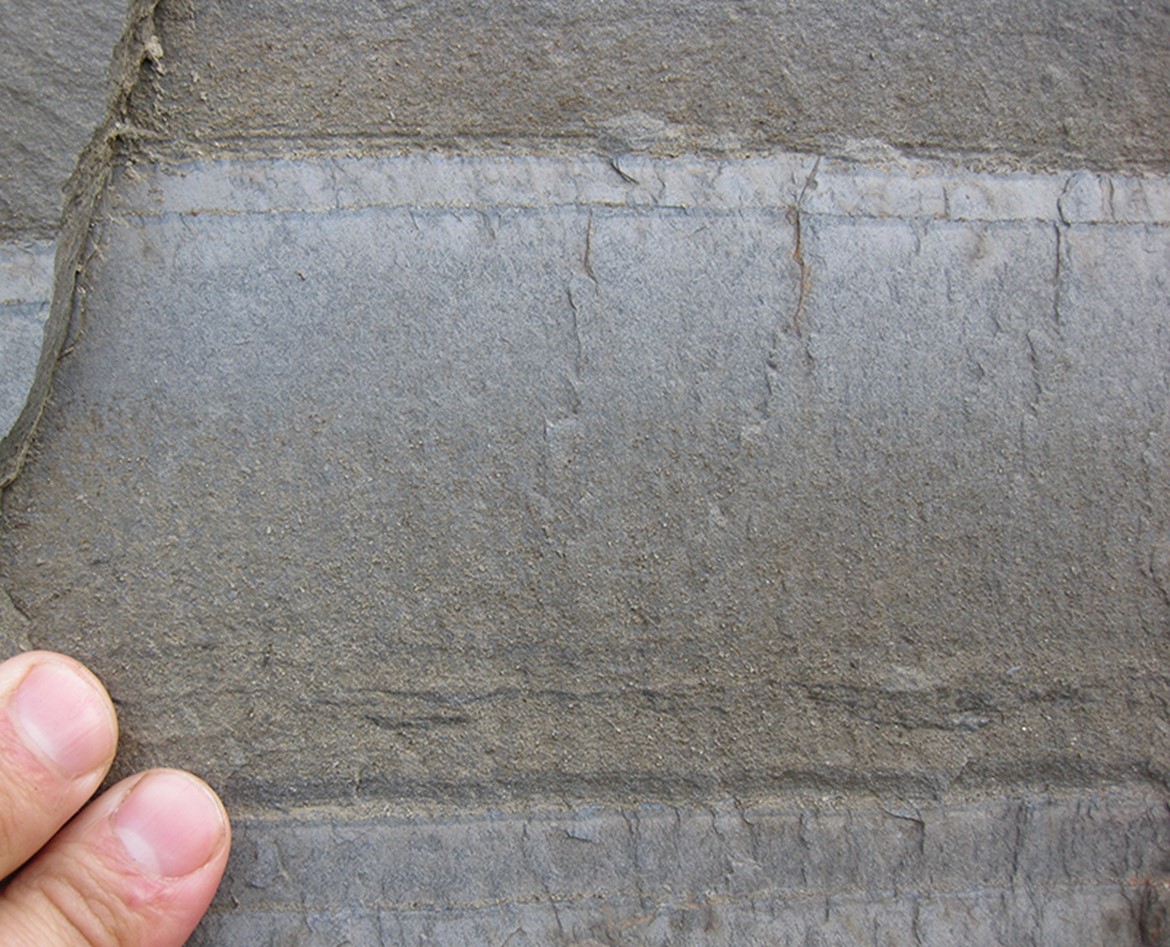
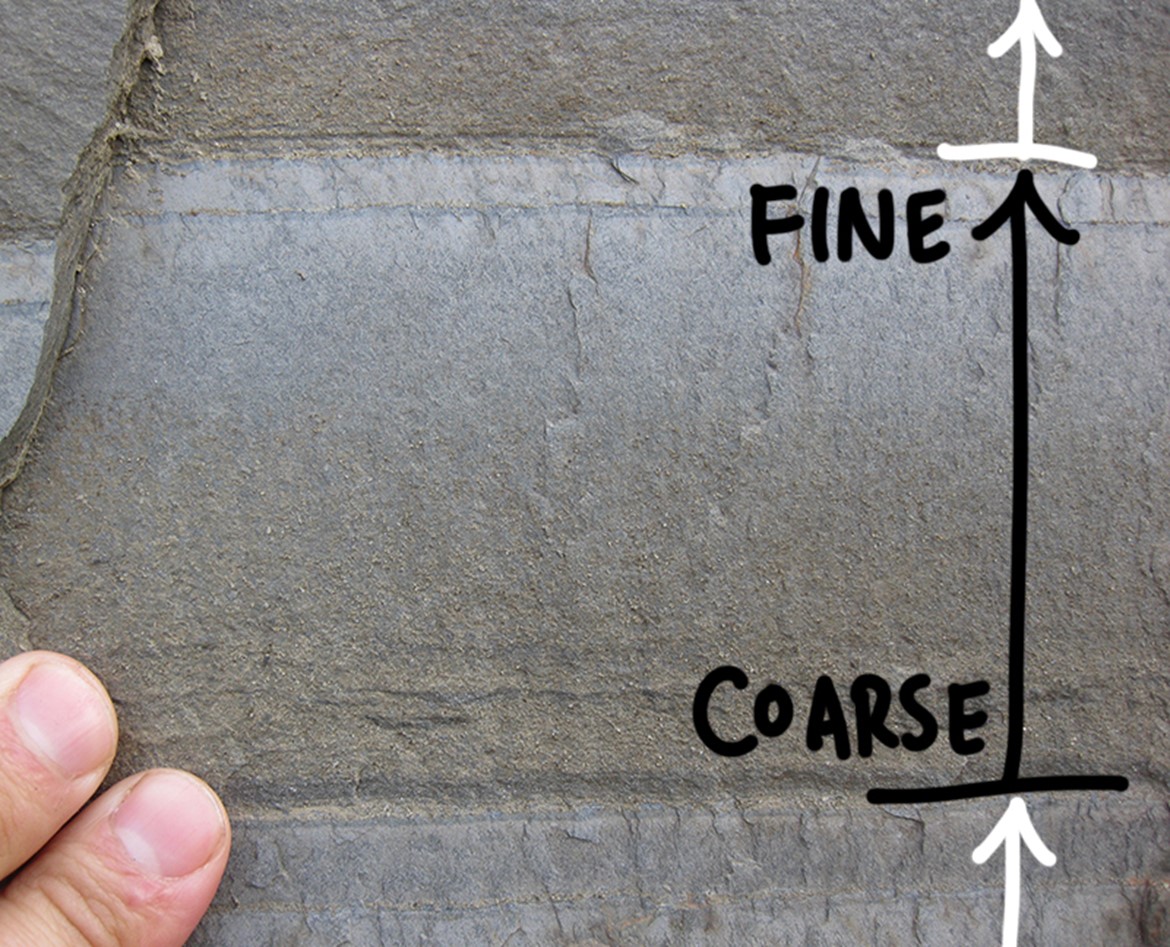
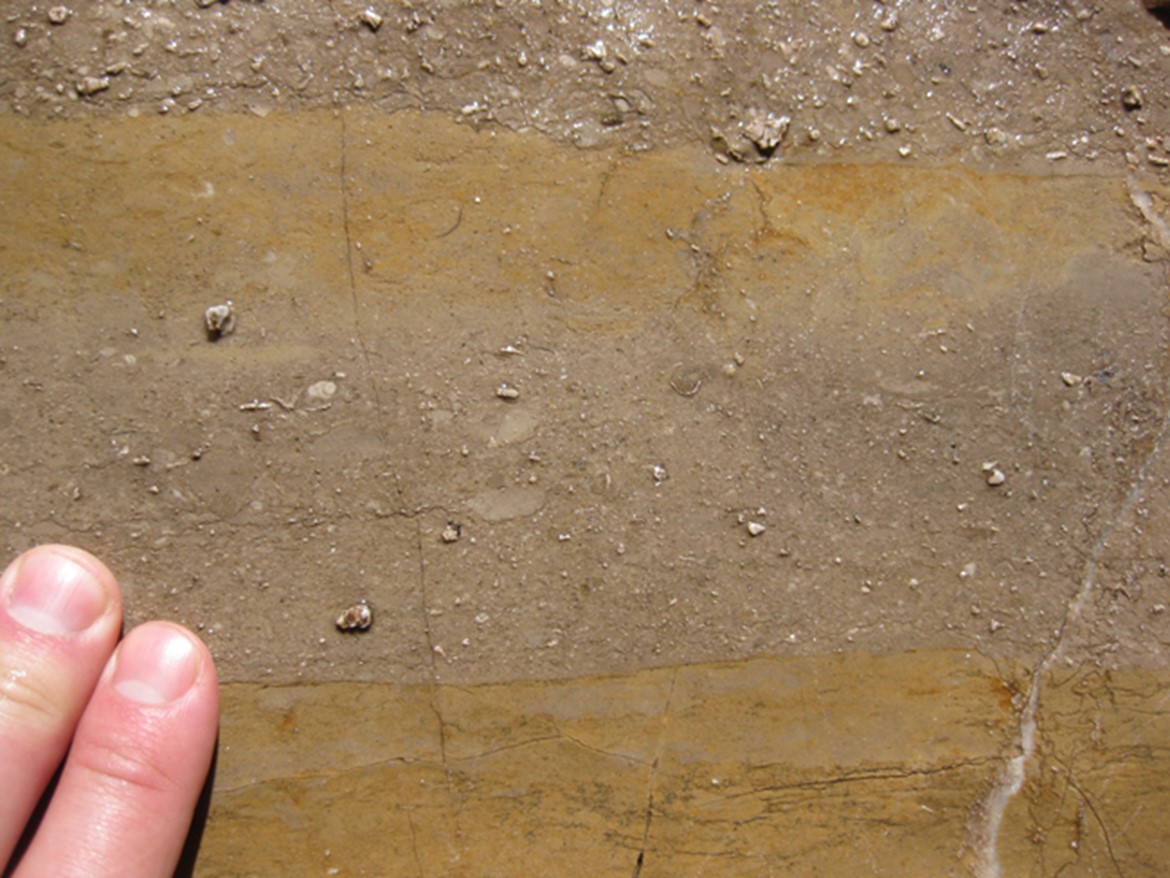
Loading structures
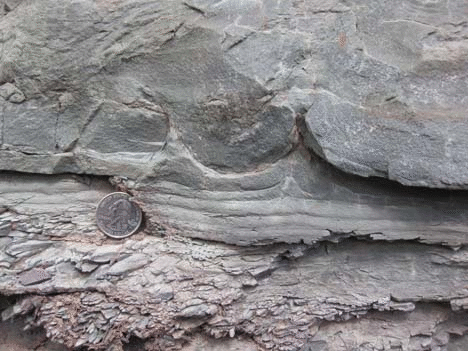
Figure \(\PageIndex{35}\): Ball & pillow structures often form along the same sand/mud interface that produces flame structures. White arrows show downward motion; red arrows show upward motion.
Loading structures form due to density inversions, when heavy wet sand is deposited suddenly atop squishy waterlogged mud. The sand sags downward in broad lobes, and the mud squirts out of the way between those lobes. Once lithified, these bulging sandy lobes and pointy shale fingers serve as excellent way-up indicators. They are best viewed in cross-section, as seen here.
Ball & pillow
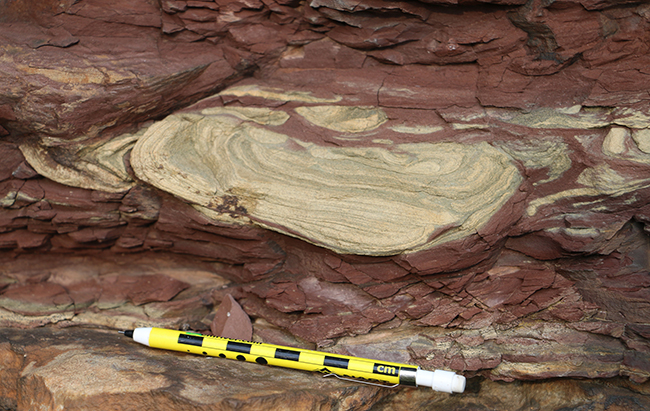
Figure \(\PageIndex{36}\): Ball & pillow loading structures made when sand was deposited on red mud, Graafwater Formation, Table Mountain, South Africa.
We call the sandy lobes that are broad and curved “ball & pillow.” These lumps make blob-like shapes that bulge downward, pointing toward gravity’s paleo-pull direction. They are lobate in form: convex downward, and concave upward. Any internal structures within the sand body, such as laminations or crossbeds, are likewise distorted downward most in the middle and least at the edges, resulting in a “smiley face” appearance in cross-section.
Here is a 3D model of some ball & pillow that formed in Devonian-aged redbeds of the Hampshire Formation in the Valley & Ridge province of West Virginia, when a thick layer of sand (today sandstone) was laid down atop a squishy layer of waterlogged mud (today red shale):
Flame structures

Figure \(\PageIndex{37}\): Flame structures in the Gualala Formation of California. (Photo by Brian Romans; reproduced with permission.)
Flame structures are what results when the mud squirts out of the way of the intrusion of the sagging sand lobes from above. It forms cuspate features that somewhat resemble flames, poking upward between the ball & pillow structures.
Did I Get It? - Quiz
What structure(s) can be found along the contact between the upper conglomerate and the muddy sandstone layer below it? (i.e. just above the head of the older geologist) What are the implications?
a. This contact shows ball & pillow structures (on the base of the conglomerate), and flame structures (on the top edge of the muddy sandstone). The implication is that there was sedimentary loading here, creating a density inversion, and the heavy gravel sagged down into waterlogged muddy sand, which squirted up around the lobes of the "ball & pillow" to form large flame structures.
b. The contact shows cross-beds, which imply deposition in a directional current. Based on the orientation of the cross-beds, the current must have been flowing from the left toward the right. The size of these cross-beds implies a migrating submarine dune, rather than a ripple. (Because of the size of the cobbles in the gravel, it could not be aeolian deposition.)
- Answer
-
a. This contact shows ball & pillow structures (on the base of the conglomerate), and flame structures (on the top edge of the muddy sandstone). The implication is that there was sedimentary loading here, creating a density inversion, and the heavy gravel sagged down into waterlogged muddy sand, which squirted up around the lobes of the "ball & pillow" to form large flame structures.
Which way is up in this outcrop? Why?
a. Down is paleo-"up," since the beds get coarser upward rather than downward, and also because of the one layer that shows up-side-down flame structures in the mud, in between upward-bulging ball & pillow structures made of sand. The beds have therefore been tectonically inverted.
b. Up is paleo-"up," since the beds get finer upward, and also because of the one layer that shows flame structures in the mud, pointing upward in between downward-bulging ball & pillow structures made of sand. The beds are therefore in their original depositional orientation.
- Answer
-
a. Down is paleo-"up," since the beds get coarser upward rather than downward, and also because of the one layer that shows up-side-down flame structures in the mud, in between upward-bulging ball & pillow structures made of sand. The beds have therefore been tectonically inverted.
Which way-up structure is shown in this 3D model?
a. Graded bedding
b. Ball & pillow
c. Cross-bedding
d. Mud cracks
- Answer
-
b. Ball & pillow
Identify the way-up structure in this outcrop, and offer an interpretation.
a. The central sandstone layer shows "ball & pillow" load structures on its lower surface. Because they are convex-downward, the strata appear to be in their original upright position. Paleo-"up" is toward the top of the photo.
b. The central sandstone layer shows cross-bedding. Because the crossbeds are tangential at the bottom and truncated at the top, the strata appear to be in their original upright position. Paleo-"up" is toward the top of the photo.
- Answer
-
a. The central sandstone layer shows "ball & pillow" load structures on its lower surface. Because they are convex-downward, the strata appear to be in their original upright position. Paleo-"up" is toward the top of the photo.
Sole structures
Sole structures are erosional features formed along the sedimentary interface by powerful currents that then deposit a bed atop their erosional divots and carvings. They include flutes, tool marks, scours, and gutters. All share in common their mode of formation: (1) there must be a pre-existing sedimentary substrate, which (2) gets eroded, making a hole or depression, and then (3) the hole gets filled in with an overlying deposit of sediment. Most all sole structures are preserved not as their original negative shape (a hole) but as the positive shape that results when that hole is filled (a cast). Let’s examine this relationship in the context of flute marks first.
Flutes & flute casts

Figure \(\PageIndex{38}\): Flute casts on the underside of a sandstone bed. Devonian of Pennsylvania.
Often the erosion takes place on/in a substrate of mud (a calm-water deposit), when current energy later increases. The increased current cuts into the soft mud, but also brings in sand (a coarser sized particle). As current energy wanes, the sand is deposited atop the mud, including in the new holes and grooves, making “casts” of them. Much later, when the sediment has all been lithified and the rock has been uplifted to Earth’s surface, the two different rock types react differently to weathering. The mud has turned to shale, which rapidly degrades and falls apart. But the sand has turned to sandstone, which typically stands up much more sturdily to the forces of weathering. It resists degradation. The result is that we typically find the bulging cast rather than the original concavity that was eroded out as a hole.

Figure \(\PageIndex{39}\): The relationship between a “negative” flute cut into mud (later lithified to shale) and the “positive” flute cast at the base of an overlying sand deposit (later lithified to sandstone).
One way of describing the situation is like this: the scour is a “negative” space (a hole), while the cast is a “positive” feature, poking out as a bulge or bump. Whether we’re lucky enough to see the original flute, or only the secondary cast of that flute, the relationship is plain. Negative spaces are cut into the paleo-“down” direction, and their infilling (the cast) mimics this same direction.
Because both the concave-up flutes and their convex-down casts bulge in the direction of paleo-“down,” they are useful as way-up indicators.
Here is a 3D model of flute casts, showing the underside of the sand bed that filled in the original flute:
Here are a few more examples to scroll through:

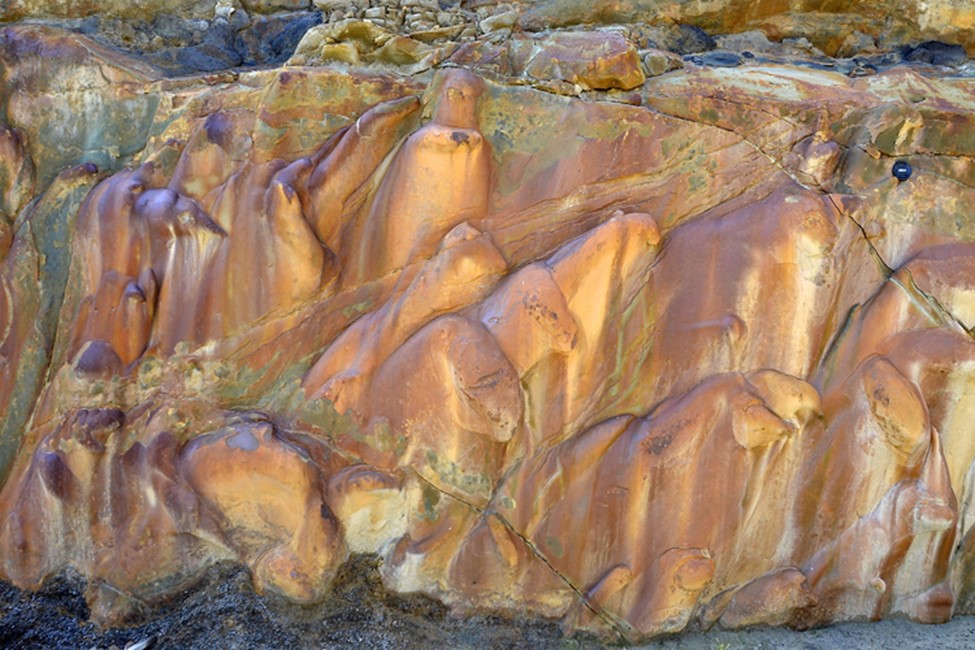
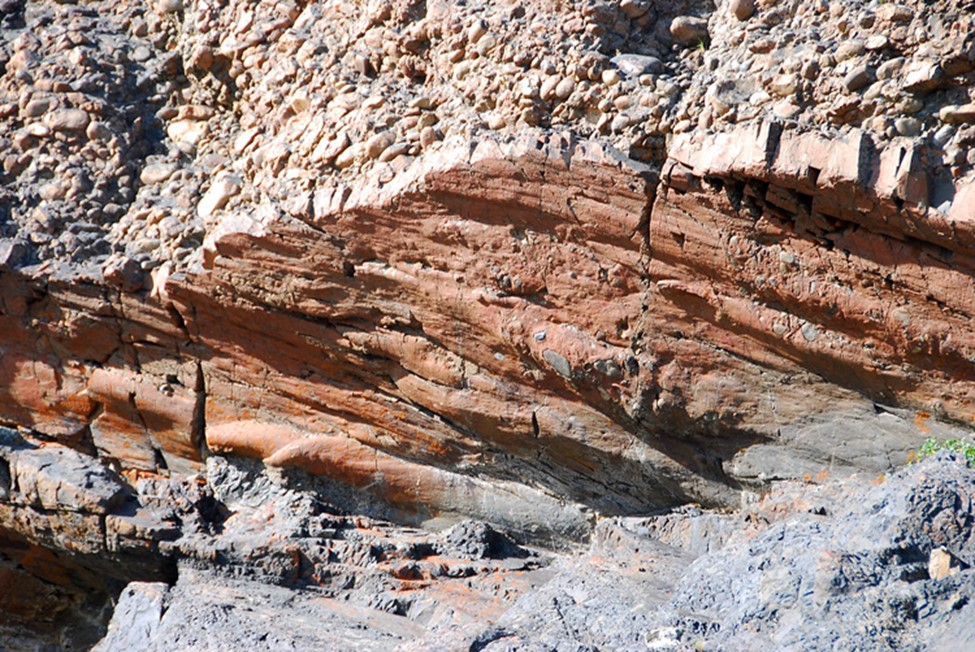
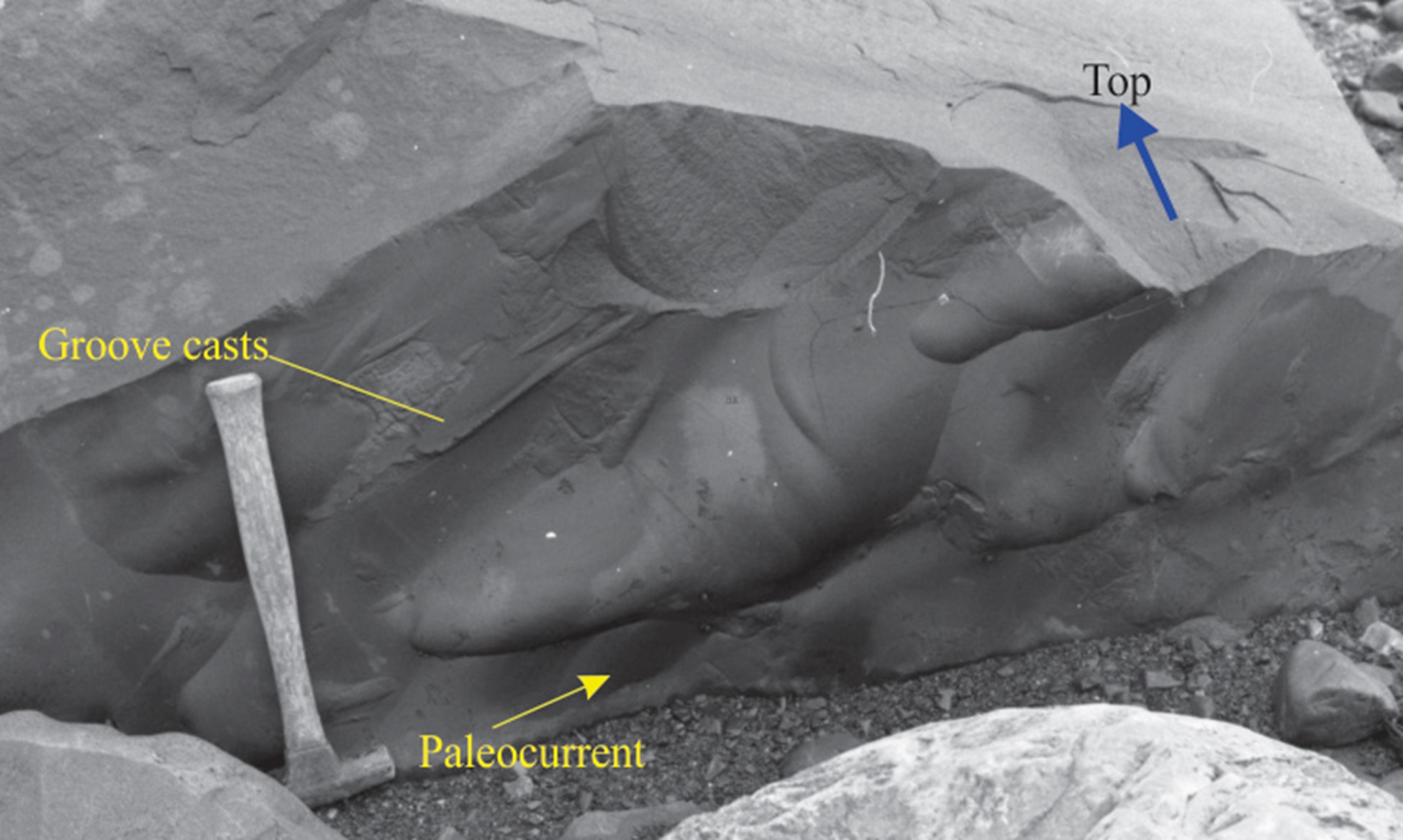
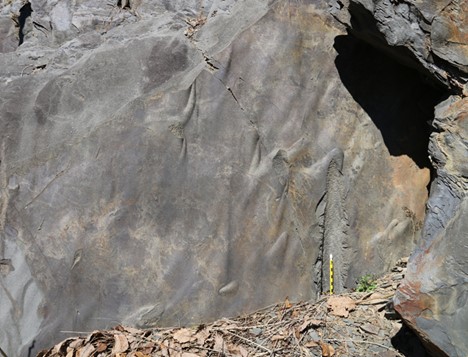
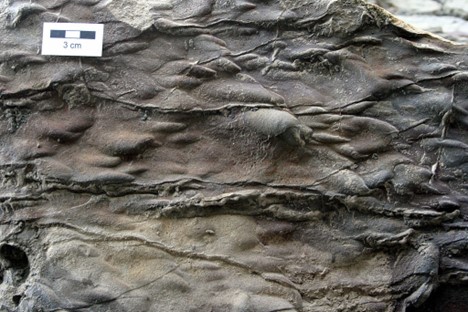
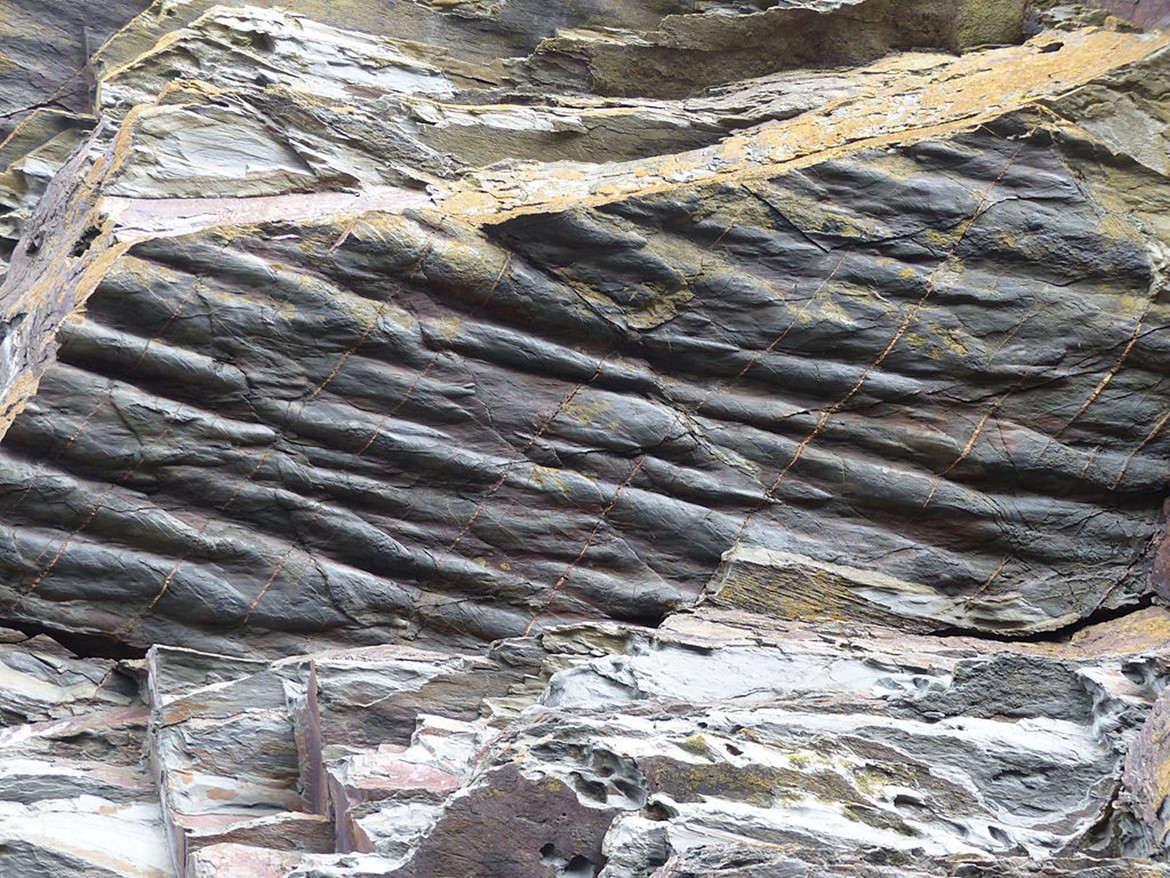

Tool marks
Tool marks form when hard objects such as cobbles, bones, wood, or shells get bounced along the bottom of a body of water, carried along by a forceful current. As the object bangs, crashes, drags, and bounces, it leaves behind a series of scratches and divots in the pre-existing sedimentary substrate. Later, these holes can be filled in with subsequent deposits, lithified, and preserved in the geologic record.
Tool marks (and casts thereof) are similar to flutes in their way-up implications: they project downward into the pre-existing sedimentary substrate, and when they are filled in by subsequent deposition, their shape is preserved as a cast on the bottom of the overlying bed. Here is an example, looking “up” at vertical Spray River Group siliciclastic strata from “below.” As such, the tool marks project off the bottom of the bed, toward our viewing perspective:
Did I Get It? - Quiz
Inspect this 3D model: Which way-up structure is shown? What are the implications?
a. This sample shows mud cracks on its upper surface; therefore this is the underside of a bed which filled in mud cracks that cut into a previously existing muddy substrate (now removed by erosion).
b. This sample shows flame structures on its upper surface; therefore this is the top side of a bed which was squished by heavier sediment being dumped on top of it (a layer now removed by erosion).
c. This sample shows flute casts on its upper surface; therefore this is the underside of a bed which filled in flutes that were cut into a previously existing substrate (now removed by erosion).
- Answer
-
c. This sample shows flute casts on its upper surface; therefore this is the underside of a bed which filled in flutes that were cut into a previously existing substrate (now removed by erosion).
Which way-up structure is this? Which way is up?
a. This sample shows casts of tool marks. We are therefore looking at the bottom of the bed.
b. This sample shows mud cracks. We are therefore looking at the bottom of the bed.
c. This sample shows casts of mud cracks. We are therefore looking at the top of the bed.
d. This sample shows tool marks. We are therefore looking at the top of the bed.
- Answer
-
a. This sample shows casts of tool marks. We are therefore looking at the bottom of the bed.
Identify the way-up structure shown in circled region of this sample (presented as a gigapixel panorama). What are the implications?
a. Three flutes are shown. The implication is that the current which deposited this bed was traveling from the right to the left, and this is the top of the resulting bed. So paleo-"up" is toward our perspective; we are looking "stratigraphically downward."
b. Two flute casts are shown. The implication is that the current which deposited this bed was traveling from the left to the right, and this is the bottom of the resulting bed. So paleo-"up" is away from our perspective; we are looking "stratigraphically upward."
c. A ball & pillow loading structure is shown. The implication is that the current which deposited this bed deposited a lot of heavy sediment quickly, and this is the bottom of the resulting bed. So paleo-"up" is away from our perspective; we are looking "stratigraphically upward."
d. Fifteen mud cracks are shown. The implication is that the sediment dried out in the open air, and this is the top of the resulting bed. So paleo-"up" is toward our perspective; we are looking "stratigraphically downward."
- Answer
-
b. Two flute casts are shown. The implication is that the current which deposited this bed was traveling from the left to the right, and this is the bottom of the resulting bed. So paleo-"up" is away from our perspective; we are looking "stratigraphically upward."
Trace fossils
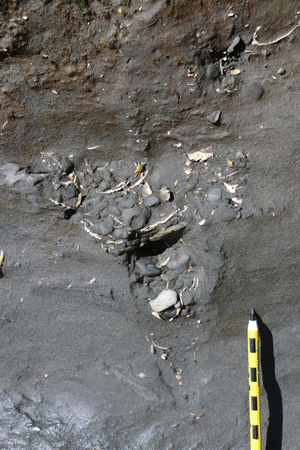
A similar logic applies to burrows, the holes dug by organisms into sedimentary substrates. An animal can only dig a hole in the mud if the mud is already there to dig in. The hole cannot precede the material into which the hole is dug. So open burrows that are later filled in with contrasting sediment can also serve as a geopetal indicator. Consider the example at right.
Similar, plants cannot take root in soil that isn’t yet there, so root traces also must penetrated in the paleo-“down” direction, at least in the most broad view. Once roots get deep enough, they can of course grow laterally or even upward in addition to growing downward.
The same idea applies to footprints, which can only press downward into extant sediment, not push upward into sedimentary deposits that haven’t yet been deposited. There are thousands of examples, but let’s consider two here: Consider the images below.
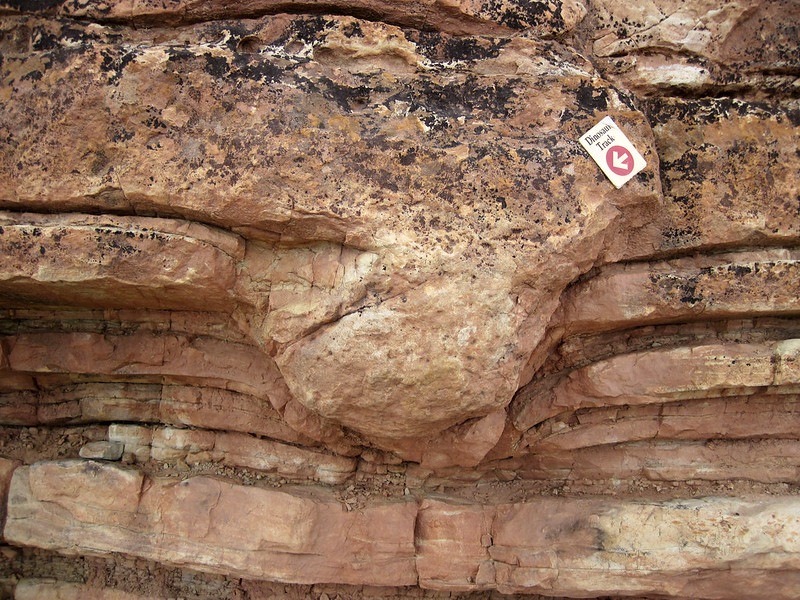
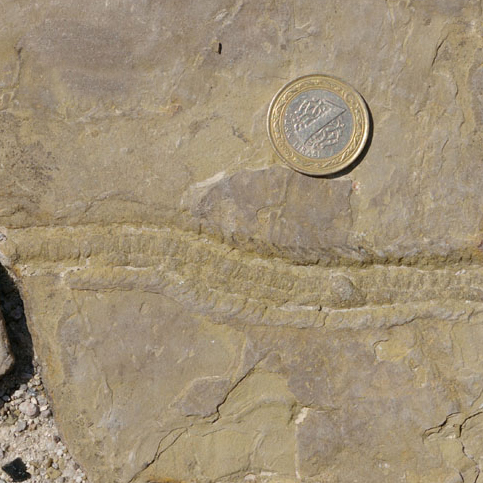
One image shows a side-view of a sauropod dinosaur track in disrupting sandstone and shale layers. The other photo shows the sinuous trackway left by some kind of invertebrate in silt, looking down on the bedding plane. We have two different perspectives here, and two different organisms, but what is in common to these bedding-surface traces is that they push/erode down into the pre-existing sedimentary substrate.
Here is a 3D model of the enigmatic Cambrian trace fossil Climactichnites, on display on a wall of a museum at the University of Wisconsin:
Note that we must be looking down on this trace, since it cuts across the ripples (and not the other way around). So this is the top of the bed of sandstone including the trace fossil, and the trace projects downward into the sand, disrupting its previous surface feature (the ripples).
The opposite is true here:
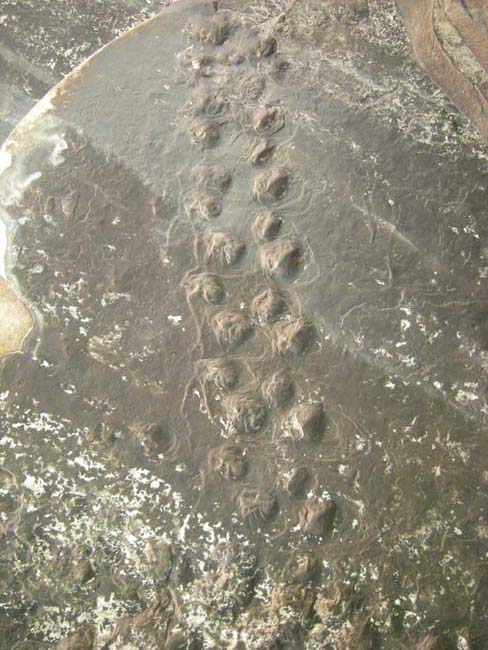
Here, in a Permian-aged reptile trackway from the Grand Canyon, the footprints poke upward from the surface of the slab of rock, implying that this is a cast of the reptile’s footprints, not the original divots pushed downward into the sediment.
Whether a monster ancient slug or a more familiar reptile, all organisms leave tracks that push downward into the sedimentary substrate over which they move. As with sole structures, the concave ‘negative space’ pokes downward into the older sedimentary layer, and the infilling creates a convex ‘positive space’ feature that projects downward off the bottom of the younger sedimentary layer.
Did I Get It? - Quiz
This 3D model shows the tracks of a giant eurypterid (sea scorpion) on a vertical sandstone bed in Scotland. What is our perspective on the orientation of the bed? Explain.
a. We are looking at the BOTTOM of the bed, since the tracks poke out toward our perspective.
b. We are looking at the TOP of the bed, since the tracks poke downward into the sandstone, away from our perspective.
c. We are looking at a CROSS-SECTIONAL view of the bed, since we can see how the tracks poke down into the sandstone toward the bottom of the screen.
- Answer
-
a. We are looking at the BOTTOM of the bed, since the tracks poke out toward our perspective.
Are we looking at the top or the bottom of this bed of Jurassic sandstone in Colorado?
a. Bottom, since the dinosaur footprints project outward from the bed's surface as casts of the original footprints.
b. Top, since the dinosaur footprints are pressed downward into the bed's surface, making "negative space" hollows.
- Answer
-
b. Top, since the dinosaur footprints are pressed downward into the bed's surface, making "negative space" hollows.
This photograph shows a bedding surface in a dune sandstone. It is lit from the upper left. What surface of the bed is this, and why?
a. It is the top of the bed, since the fossil footprints are depressed into the surface, making hollow holes.
b. It is a cross-section of the bed, since we can see a sideways-view of the footprint shape.
c. It is the underside of the bed, since casts of the fossil footprints poke out of its surface.
- Answer
-
c. It is the underside of the bed, since casts of the fossil footprints poke out of its surface.
Are we looking at the top or the bottom of this bed of late Cretaceous limestone in Bolivia?
a. Bottom, since the dinosaur footprints project outward from the bed's surface as casts of the original footprints.
b. Top, since the dinosaur footprints are pressed downward into the bed's surface, making "negative space" hollows.
- Answer
-
b. Top, since the dinosaur footprints are pressed downward into the bed's surface, making "negative space" hollows.
Are we looking at the top or the bottom of this bed of Cambrian sandstone in Arizona?
a. Bottom, since the trace fossil pokes out at us as a positive-weathering feature. This is a cast of a trilobite crawling trace.
b. Top, since the trace fossil is depressed as a hollow (or "negative space") feature on the surface of the bed.
- Answer
-
a. Bottom, since the trace fossil pokes out at us as a positive-weathering feature. This is a cast of a trilobite crawling trace.
Which way is the geologist pointing? Why?
a. The geologist's hand is pointing downward, showing the direction a dinosaur's foot squished into sedimentary layers. Later, the footprint was filled in with a massive sand layer, preserving the trace fossil as a cast.
b. The geologist is pointing in the paleo-"up" direction, showing an up-side-down stromatolite. It bulges downward (convex down), which means these strata must have been tectonically inverted.
- Answer
-
a. The geologist's hand is pointing downward, showing the direction a dinosaur's foot squished into sedimentary layers. Later, the footprint was filled in with a massive sand layer, preserving the trace fossil as a cast.
Stromatolites

Stromatolites are layered microbial mats, common in Precambrian shallow-water sediments, particularly carbonates, but also sometimes siliciclastic sediments or even banded iron formations. Their photosynthetic life habit causes them to grow upward toward the sunlight, giving them convex-upward (dome-like) shapes. If concave-up instead, they can be interpreted to be up-side-down.
Here is a gigapixel panorama of an outcrop of stromatolites in Banff National Park, Alberta. Explore it to get a sense of their shape when right-side-up:
Here is a 3D model of a stromatolite, from the Mesoproterozoic Belt Supergroup of Montana. Rotate it around to get a sense of how the cross-sectional views on each side relate to the 3D dome-like structure of the fossil (visible on top):
Microbial mats can take on many shapes and textures, but this upward-doming shape of stromatolites is a reliable way-up indicator, especially when there are many individual stromatolites all pointing the same direction.
Test your identification of stromatolite younging direction with this self-quiz:
Did I Get It? - Quiz
Identify which way-up structure is shown in this vertical outcrop face. Are the strata right-side-up, or in some other orientation?
a. There are numerous cross-beds shown here. Because the cross-beds show tangential bottoms at the bottom of the photo and truncated upper surfaces toward the top of the photo (i.e. they are concave-up), they must be in their original depositional orientation.
b. There are numerous stromatolites shown here. Because the stromatolites dome downward toward the bottom of the photo (i.e. they are concave-up), they must be tectonically inverted.
c. There are numerous stromatolites shown here. Because the stromatolites dome upward toward the top of the photo (i.e. they are convex-up), they must be in their original depositional orientation.
d. There are numerous cross-beds shown here. Because the cross-beds show tangential contacts with the main bed at the top of the photo and truncated surfaces toward the bottom of the photo (i.e. they are concave-down), they must be tectonically inverted.
- Answer
-
c. There are numerous stromatolites shown here. Because the stromatolites dome upward toward the top of the photo (i.e. they are convex-up), they must be in their original depositional orientation.
Examine this gigapixel panorama of a boulder of limestone containing stromatolites. Which way is paleo-"up," and why?
a. Left is the paleo-"up" direction, since the stromatolites' convex shapes dome in that direction.
b. Down is the paleo-"up" direction, since the stromatolites' convex shapes dome in that direction.
c. Up is the paleo-"up" direction, since the stromatolites' convex shapes dome in that direction.
- Answer
-
b. Down is the paleo-"up" direction, since the stromatolites' convex shapes dome in that direction.
Examine this 3D model of a sample showing a series of small stromatolites. Which way is the paleo-"up" direction, and why?
a. As the model first loaded up, LEFT is the paleo-"up" direction, since the stromatolites' convex shapes dome in that direction.
b. As the model first loaded up, DOWN is the paleo-"up" direction, since the stromatolites' convex shapes dome in that direction.
c. As the model first loaded up, UP is the paleo-"up" direction, since the stromatolites' convex shapes dome in that direction.
- Answer
-
a. As the model first loaded up, LEFT is the paleo-"up" direction, since the stromatolites' convex shapes dome in that direction.