4.4: The Chemistry of Minerals
- Page ID
- 22621
\( \newcommand{\vecs}[1]{\overset { \scriptstyle \rightharpoonup} {\mathbf{#1}} } \)
\( \newcommand{\vecd}[1]{\overset{-\!-\!\rightharpoonup}{\vphantom{a}\smash {#1}}} \)
\( \newcommand{\id}{\mathrm{id}}\) \( \newcommand{\Span}{\mathrm{span}}\)
( \newcommand{\kernel}{\mathrm{null}\,}\) \( \newcommand{\range}{\mathrm{range}\,}\)
\( \newcommand{\RealPart}{\mathrm{Re}}\) \( \newcommand{\ImaginaryPart}{\mathrm{Im}}\)
\( \newcommand{\Argument}{\mathrm{Arg}}\) \( \newcommand{\norm}[1]{\| #1 \|}\)
\( \newcommand{\inner}[2]{\langle #1, #2 \rangle}\)
\( \newcommand{\Span}{\mathrm{span}}\)
\( \newcommand{\id}{\mathrm{id}}\)
\( \newcommand{\Span}{\mathrm{span}}\)
\( \newcommand{\kernel}{\mathrm{null}\,}\)
\( \newcommand{\range}{\mathrm{range}\,}\)
\( \newcommand{\RealPart}{\mathrm{Re}}\)
\( \newcommand{\ImaginaryPart}{\mathrm{Im}}\)
\( \newcommand{\Argument}{\mathrm{Arg}}\)
\( \newcommand{\norm}[1]{\| #1 \|}\)
\( \newcommand{\inner}[2]{\langle #1, #2 \rangle}\)
\( \newcommand{\Span}{\mathrm{span}}\) \( \newcommand{\AA}{\unicode[.8,0]{x212B}}\)
\( \newcommand{\vectorA}[1]{\vec{#1}} % arrow\)
\( \newcommand{\vectorAt}[1]{\vec{\text{#1}}} % arrow\)
\( \newcommand{\vectorB}[1]{\overset { \scriptstyle \rightharpoonup} {\mathbf{#1}} } \)
\( \newcommand{\vectorC}[1]{\textbf{#1}} \)
\( \newcommand{\vectorD}[1]{\overrightarrow{#1}} \)
\( \newcommand{\vectorDt}[1]{\overrightarrow{\text{#1}}} \)
\( \newcommand{\vectE}[1]{\overset{-\!-\!\rightharpoonup}{\vphantom{a}\smash{\mathbf {#1}}}} \)
\( \newcommand{\vecs}[1]{\overset { \scriptstyle \rightharpoonup} {\mathbf{#1}} } \)
\( \newcommand{\vecd}[1]{\overset{-\!-\!\rightharpoonup}{\vphantom{a}\smash {#1}}} \)
\(\newcommand{\avec}{\mathbf a}\) \(\newcommand{\bvec}{\mathbf b}\) \(\newcommand{\cvec}{\mathbf c}\) \(\newcommand{\dvec}{\mathbf d}\) \(\newcommand{\dtil}{\widetilde{\mathbf d}}\) \(\newcommand{\evec}{\mathbf e}\) \(\newcommand{\fvec}{\mathbf f}\) \(\newcommand{\nvec}{\mathbf n}\) \(\newcommand{\pvec}{\mathbf p}\) \(\newcommand{\qvec}{\mathbf q}\) \(\newcommand{\svec}{\mathbf s}\) \(\newcommand{\tvec}{\mathbf t}\) \(\newcommand{\uvec}{\mathbf u}\) \(\newcommand{\vvec}{\mathbf v}\) \(\newcommand{\wvec}{\mathbf w}\) \(\newcommand{\xvec}{\mathbf x}\) \(\newcommand{\yvec}{\mathbf y}\) \(\newcommand{\zvec}{\mathbf z}\) \(\newcommand{\rvec}{\mathbf r}\) \(\newcommand{\mvec}{\mathbf m}\) \(\newcommand{\zerovec}{\mathbf 0}\) \(\newcommand{\onevec}{\mathbf 1}\) \(\newcommand{\real}{\mathbb R}\) \(\newcommand{\twovec}[2]{\left[\begin{array}{r}#1 \\ #2 \end{array}\right]}\) \(\newcommand{\ctwovec}[2]{\left[\begin{array}{c}#1 \\ #2 \end{array}\right]}\) \(\newcommand{\threevec}[3]{\left[\begin{array}{r}#1 \\ #2 \\ #3 \end{array}\right]}\) \(\newcommand{\cthreevec}[3]{\left[\begin{array}{c}#1 \\ #2 \\ #3 \end{array}\right]}\) \(\newcommand{\fourvec}[4]{\left[\begin{array}{r}#1 \\ #2 \\ #3 \\ #4 \end{array}\right]}\) \(\newcommand{\cfourvec}[4]{\left[\begin{array}{c}#1 \\ #2 \\ #3 \\ #4 \end{array}\right]}\) \(\newcommand{\fivevec}[5]{\left[\begin{array}{r}#1 \\ #2 \\ #3 \\ #4 \\ #5 \\ \end{array}\right]}\) \(\newcommand{\cfivevec}[5]{\left[\begin{array}{c}#1 \\ #2 \\ #3 \\ #4 \\ #5 \\ \end{array}\right]}\) \(\newcommand{\mattwo}[4]{\left[\begin{array}{rr}#1 \amp #2 \\ #3 \amp #4 \\ \end{array}\right]}\) \(\newcommand{\laspan}[1]{\text{Span}\{#1\}}\) \(\newcommand{\bcal}{\cal B}\) \(\newcommand{\ccal}{\cal C}\) \(\newcommand{\scal}{\cal S}\) \(\newcommand{\wcal}{\cal W}\) \(\newcommand{\ecal}{\cal E}\) \(\newcommand{\coords}[2]{\left\{#1\right\}_{#2}}\) \(\newcommand{\gray}[1]{\color{gray}{#1}}\) \(\newcommand{\lgray}[1]{\color{lightgray}{#1}}\) \(\newcommand{\rank}{\operatorname{rank}}\) \(\newcommand{\row}{\text{Row}}\) \(\newcommand{\col}{\text{Col}}\) \(\renewcommand{\row}{\text{Row}}\) \(\newcommand{\nul}{\text{Nul}}\) \(\newcommand{\var}{\text{Var}}\) \(\newcommand{\corr}{\text{corr}}\) \(\newcommand{\len}[1]{\left|#1\right|}\) \(\newcommand{\bbar}{\overline{\bvec}}\) \(\newcommand{\bhat}{\widehat{\bvec}}\) \(\newcommand{\bperp}{\bvec^\perp}\) \(\newcommand{\xhat}{\widehat{\xvec}}\) \(\newcommand{\vhat}{\widehat{\vvec}}\) \(\newcommand{\uhat}{\widehat{\uvec}}\) \(\newcommand{\what}{\widehat{\wvec}}\) \(\newcommand{\Sighat}{\widehat{\Sigma}}\) \(\newcommand{\lt}{<}\) \(\newcommand{\gt}{>}\) \(\newcommand{\amp}{&}\) \(\definecolor{fillinmathshade}{gray}{0.9}\)Rocks are composed of minerals that have a specific chemical composition. To understand mineral chemistry, it is essential to examine the fundamental unit of all matter, the atom.
Atoms
Matter is made of atoms. Atoms consist of subatomic particles: protons, neutrons, and electrons. A simple model of the atom has a central nucleus composed of protons, which have a positive charge, and neutrons which have no charge. A cloud of negatively charged electrons surrounds the nucleus, with the number of electrons equaling the number of protons, balancing with the positive charge of the protons for a neutral atom. Protons and neutrons each have a mass number of 1. The mass of an electron is less than 1/1000th that of a proton or neutron, meaning most of the atom’s mass is in the nucleus. For our purposes, we can simplify the math by rounding the electron’s mass down to zero.
The Periodic Table of Elements
Matter is composed of elements which are atoms that have a specific number of protons in the nucleus. This number of protons is called the atomic number of the element. For example, an oxygen atom has 8 protons and an iron atom has 26 protons. An element cannot be broken down chemically into a simpler form - they are ‘elemental’ for this reason! (Of course, nuclear reactions can break an atom down to a smaller, simpler form, but nuclear reactions are not chemical reactions). Down to the atom, each element retains unique chemical and physical properties, leading to particular behaviors in nature. This uniqueness led scientists to develop a periodic table of the elements, a tabular arrangement of all known elements listed in order of their atomic number and chemical properties.

The first arrangement of elements into a periodic table was done by Dmitri Mendeleev in 1869 using the elements known at the time [1]. In the periodic table, each element has a chemical symbol, name, atomic number, and atomic mass. The chemical symbol is an abbreviation for the element, often derived from a Latin or Greek name for the substance (for example, lead is Pb from the Latin ‘plumbum’) [2]. The atomic number is the number of protons in the nucleus. The atomic mass is the number of protons and neutrons in the nucleus, each with a mass number of one. Since the mass of electrons is so much less than that of protons and neutrons, the atomic mass is effectively the number of protons plus the number of neutrons. For example, the element silicon (Si) is atomic number 14 on the periodic table. Silicon has 14 protons and 14 neutrons in its nucleus and 14 electrons located in its electron cloud. The atomic mass of silicon is 28.01 mass units.
Isotopes
The atomic mass of an element represents an average mass of all of the atoms of that element found in nature. The atomic mass, noted on the periodic table, is usually not a whole number. Atoms of the same element can have differing numbers of neutrons in their nucleus. These are referred to as isotopes of that element. Isotopes behave the same chemically however they are slightly different in mass.
Isotopes are very important to Historical Geology. Applications of the use of isotopes in geology is discussed elsewhere in this text.
Common Elements of the Earth’s Crust
Among the 118 known elements appearing on the periodic table, the heaviest are fleeting human creations known only in high energy particle accelerators, and they decay rapidly. The naturally occurring elements on the periodic table end with uranium, atomic number 92. Of these 92 elements, only eight are abundant in the Earth’s crust and are shown in the pie chart below. Therefore, it is of these eight elements that the most common rock-forming minerals are composed.
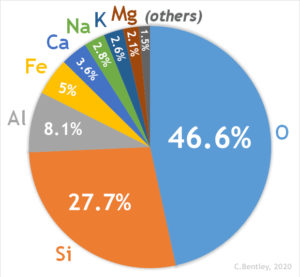
Chemical Bonding
Minerals are formed by the chemical bonding that occurs between these elements. Most minerals are compounds containing multiple elements bonded together in a specific arrangement. Chemical bonding describes how these atoms attach with each other to form compounds, such as sodium (Na) and chlorine (Cl) combine to form the mineral halite with the chemical formula: NaCl. The mineral halite is common table salt. There are some minerals that are composed of only one element, such as native Copper (Cu), native Gold (Au), or native Silver (Ag). These single elements bond to each other to form molecules, however they are not compounds because they are made of only one element.
Valence, Charge and Ions
The electrons around the atom’s nucleus are located in shells representing different energy levels. The outermost shell is called the valence shell. Electrons in the valence shell are involved in chemical bonding. In 1913, Niels Bohr (1885-1962) proposed a simple model of the atom that states atoms are more stable when their outermost shell is full [3, 4]. Atoms of most elements thus tend to gain or lose electrons so the outermost or valence shell is full. Elements that appear on the left side of the periodic table have smaller numbers of electrons in their outermost (valence) shell while elements on the right side have increasingly greater numbers of electrons in the valence shell. It takes a great deal of energy for the nucleus of an atom to hang on to a small number of valence electrons. These elements tend to search out partners that need a few electrons to fill their valence shell. If an element gains or loses electrons in its valence shell, it is then referred to as an ion. Because the number of electrons and protons are no longer equal in an ion, it has a charge. The charge is positive if protons outnumber electrons, and negative if electrons outnumber protons.
Let’s use a familiar example to help illustrate. Sodium (Na) is located on the far left side of the Periodic Table. It has one lonely electron in its valence shell that it desperately wants to shed. Remember, one atom of sodium (Na) has the same number of protons in its nucleus as it has electrons in its cloud. Therefore, if it loses any electrons it will have an excess positive charge because the positive charge of the protons in the nucleus will now outnumber the electrons. As sodium (Na) relinquishes its one valence electron is becomes a positively charged ion called a cation and is designated \(\ce{Na^+}\). Coincidentally, chlorine (Cl) has one spot left for an electron in its valence shell. If chlorine (Cl) gains an electron, it will have a net negative charge and is referred to as an anion and is designated \(\ce{Cl^-}\).


A chemical bond will form between these oppositely charged ions which creates a molecule. This type of chemical bond is an ionic bond where electron transfer and a strong attraction between oppositely charged ions creates the bond. This molecule of NaCl (sodium chloride) is not a mineral. Remember, a mineral must have an “orderly and repeating internal crystalline structure.” The smallest representation of a mineral is one unit cell. The unit cell for the mineral halite (sodium chloride, NaCl) appears in the figure below where the sodium atoms are purple and the chlorine atoms are green.
Explore the following 3D molecular crystal structure of the mineral halite, below. Roll your cursor over to grab and rotate the structure.
Now, take a look at a 3D representation of perfect mineral crystals of halite.
Do you notice the similarity of the molecular structure with the outward appearance of the crystals? The outward physical appearance of a mineral will reflect its internal atomic structure. How cool is it that the atomic level structure of a mineral is revealed in its macroscopic (hand-sample sized) shape! We will look at this in more detail in the upcoming section on diagnostic mineral characteristics.
Another type of chemical bonding can occur between elements in the formation of minerals that involves electron sharing. This is most easily explained in looking at a simple molecular model of \(\ce{H2O}\), water. Oxygen has 6 electrons in its valence shell and hydrogen has only one. By sharing these electrons, the valence shells will contain 8 electrons for oxygen and 2 for hydrogen. Both configurations are very stable.
The Silica Tetrahedron
The most common covalent bond in the formation of minerals is the bond that occurs between silicon and oxygen. The pie chart in the previous section shows us that the two most common elements in the Earth’s crust are oxygen and silicon. It makes sense that the most common minerals in the crust will therefore contain a lot of these two elements. The first nine of “The Big Ten” minerals are indeed from the largest mineral group, the silicate minerals. The silicates are named for the silicon and oxygen foundation in their composition. The basic framework of the silicate minerals is the silicon - oxygen (or silica) tetrahedron where four oxygen atoms pack very tightly around one silicon atom. The resulting molecular structure is shaped like a tetrahedron.

The silica tetrahedron is referred to as an “complex molecule.” This means that the silicon and oxygen atoms are very tightly bonded however the overall charge is negative making this complex molecule a complex ion. The silica molecules acts as an anion. The silicon atom has four valence electrons while the oxygen atoms each have six valence electrons. To fill the outermost electron shell, each atom would need to share a total of eight electrons. This cannot happen since silicon has only four electrons to share. The result is a complex molecule with a net negative charge of -4. As a result, this complex silica molecule is ready to bond with other available elements or complex molecules. This is how all of the silicate minerals form.
Let’s look at one of our “Big Ten” silicate minerals, olivine, to see how this works.

Olivine is largely an “igneous mineral” meaning that olivine most commonly forms as a magma cools and minerals begin to crystallize. The chemical formula for the mineral olivine is: \(\bf{\ce{(Mg,Fe)2SiO4}}\) The elements of Mg (magnesium) and Fe (iron) appear together in parentheses because they can readily substitute for each other in the mineral’s crystalline structure depending on which of the elements are available in the cooling magma.
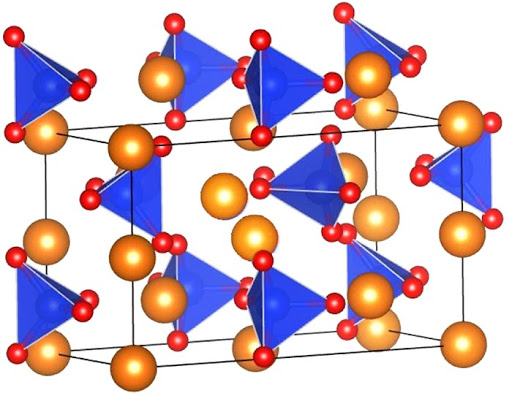
Both Mg and Fe have a valence charge of +2; both Mg and Fe have two electrons in their outermost shells, e.g. electrons that they are looking to “give away.” They are swimming around in that magma looking for partners! As the magma cools, it will reach a temperature at which bonding can occur. As discussed, the complex silica molecule forms with the overall -4 charge. The available Fe+2 and Mg+2 will form an ionic bond with the complex silica molecule which will balance out the overall charge. However, as indicated above with the example of the mineral halite, one molecule does not constitute a mineral. The smallest representation of a mineral is one unit cell which appears in the diagram (right) for olivine.
[1] Mendeleev, D. The relation between the properties and atomic weights of the elements. Journal of the Russian Chemical Society 1, 60–77 (1869)
[2] Scerri, E. R. The Periodic Table: Its Story and Its Significance. (Oxford University Press, USA, 2007)
[3] Science literacy: concepts, contexts, and consequences. (National Academies Press (US), 2016).
[4]Bohr, N. On the constitution of atoms and molecules. Philos. Mag. 26, 1–24 (1913)