7.2: Absolute Dating
- Page ID
- 28251
\( \newcommand{\vecs}[1]{\overset { \scriptstyle \rightharpoonup} {\mathbf{#1}} } \)
\( \newcommand{\vecd}[1]{\overset{-\!-\!\rightharpoonup}{\vphantom{a}\smash {#1}}} \)
\( \newcommand{\id}{\mathrm{id}}\) \( \newcommand{\Span}{\mathrm{span}}\)
( \newcommand{\kernel}{\mathrm{null}\,}\) \( \newcommand{\range}{\mathrm{range}\,}\)
\( \newcommand{\RealPart}{\mathrm{Re}}\) \( \newcommand{\ImaginaryPart}{\mathrm{Im}}\)
\( \newcommand{\Argument}{\mathrm{Arg}}\) \( \newcommand{\norm}[1]{\| #1 \|}\)
\( \newcommand{\inner}[2]{\langle #1, #2 \rangle}\)
\( \newcommand{\Span}{\mathrm{span}}\)
\( \newcommand{\id}{\mathrm{id}}\)
\( \newcommand{\Span}{\mathrm{span}}\)
\( \newcommand{\kernel}{\mathrm{null}\,}\)
\( \newcommand{\range}{\mathrm{range}\,}\)
\( \newcommand{\RealPart}{\mathrm{Re}}\)
\( \newcommand{\ImaginaryPart}{\mathrm{Im}}\)
\( \newcommand{\Argument}{\mathrm{Arg}}\)
\( \newcommand{\norm}[1]{\| #1 \|}\)
\( \newcommand{\inner}[2]{\langle #1, #2 \rangle}\)
\( \newcommand{\Span}{\mathrm{span}}\) \( \newcommand{\AA}{\unicode[.8,0]{x212B}}\)
\( \newcommand{\vectorA}[1]{\vec{#1}} % arrow\)
\( \newcommand{\vectorAt}[1]{\vec{\text{#1}}} % arrow\)
\( \newcommand{\vectorB}[1]{\overset { \scriptstyle \rightharpoonup} {\mathbf{#1}} } \)
\( \newcommand{\vectorC}[1]{\textbf{#1}} \)
\( \newcommand{\vectorD}[1]{\overrightarrow{#1}} \)
\( \newcommand{\vectorDt}[1]{\overrightarrow{\text{#1}}} \)
\( \newcommand{\vectE}[1]{\overset{-\!-\!\rightharpoonup}{\vphantom{a}\smash{\mathbf {#1}}}} \)
\( \newcommand{\vecs}[1]{\overset { \scriptstyle \rightharpoonup} {\mathbf{#1}} } \)
\( \newcommand{\vecd}[1]{\overset{-\!-\!\rightharpoonup}{\vphantom{a}\smash {#1}}} \)
\(\newcommand{\avec}{\mathbf a}\) \(\newcommand{\bvec}{\mathbf b}\) \(\newcommand{\cvec}{\mathbf c}\) \(\newcommand{\dvec}{\mathbf d}\) \(\newcommand{\dtil}{\widetilde{\mathbf d}}\) \(\newcommand{\evec}{\mathbf e}\) \(\newcommand{\fvec}{\mathbf f}\) \(\newcommand{\nvec}{\mathbf n}\) \(\newcommand{\pvec}{\mathbf p}\) \(\newcommand{\qvec}{\mathbf q}\) \(\newcommand{\svec}{\mathbf s}\) \(\newcommand{\tvec}{\mathbf t}\) \(\newcommand{\uvec}{\mathbf u}\) \(\newcommand{\vvec}{\mathbf v}\) \(\newcommand{\wvec}{\mathbf w}\) \(\newcommand{\xvec}{\mathbf x}\) \(\newcommand{\yvec}{\mathbf y}\) \(\newcommand{\zvec}{\mathbf z}\) \(\newcommand{\rvec}{\mathbf r}\) \(\newcommand{\mvec}{\mathbf m}\) \(\newcommand{\zerovec}{\mathbf 0}\) \(\newcommand{\onevec}{\mathbf 1}\) \(\newcommand{\real}{\mathbb R}\) \(\newcommand{\twovec}[2]{\left[\begin{array}{r}#1 \\ #2 \end{array}\right]}\) \(\newcommand{\ctwovec}[2]{\left[\begin{array}{c}#1 \\ #2 \end{array}\right]}\) \(\newcommand{\threevec}[3]{\left[\begin{array}{r}#1 \\ #2 \\ #3 \end{array}\right]}\) \(\newcommand{\cthreevec}[3]{\left[\begin{array}{c}#1 \\ #2 \\ #3 \end{array}\right]}\) \(\newcommand{\fourvec}[4]{\left[\begin{array}{r}#1 \\ #2 \\ #3 \\ #4 \end{array}\right]}\) \(\newcommand{\cfourvec}[4]{\left[\begin{array}{c}#1 \\ #2 \\ #3 \\ #4 \end{array}\right]}\) \(\newcommand{\fivevec}[5]{\left[\begin{array}{r}#1 \\ #2 \\ #3 \\ #4 \\ #5 \\ \end{array}\right]}\) \(\newcommand{\cfivevec}[5]{\left[\begin{array}{c}#1 \\ #2 \\ #3 \\ #4 \\ #5 \\ \end{array}\right]}\) \(\newcommand{\mattwo}[4]{\left[\begin{array}{rr}#1 \amp #2 \\ #3 \amp #4 \\ \end{array}\right]}\) \(\newcommand{\laspan}[1]{\text{Span}\{#1\}}\) \(\newcommand{\bcal}{\cal B}\) \(\newcommand{\ccal}{\cal C}\) \(\newcommand{\scal}{\cal S}\) \(\newcommand{\wcal}{\cal W}\) \(\newcommand{\ecal}{\cal E}\) \(\newcommand{\coords}[2]{\left\{#1\right\}_{#2}}\) \(\newcommand{\gray}[1]{\color{gray}{#1}}\) \(\newcommand{\lgray}[1]{\color{lightgray}{#1}}\) \(\newcommand{\rank}{\operatorname{rank}}\) \(\newcommand{\row}{\text{Row}}\) \(\newcommand{\col}{\text{Col}}\) \(\renewcommand{\row}{\text{Row}}\) \(\newcommand{\nul}{\text{Nul}}\) \(\newcommand{\var}{\text{Var}}\) \(\newcommand{\corr}{\text{corr}}\) \(\newcommand{\len}[1]{\left|#1\right|}\) \(\newcommand{\bbar}{\overline{\bvec}}\) \(\newcommand{\bhat}{\widehat{\bvec}}\) \(\newcommand{\bperp}{\bvec^\perp}\) \(\newcommand{\xhat}{\widehat{\xvec}}\) \(\newcommand{\vhat}{\widehat{\vvec}}\) \(\newcommand{\uhat}{\widehat{\uvec}}\) \(\newcommand{\what}{\widehat{\wvec}}\) \(\newcommand{\Sighat}{\widehat{\Sigma}}\) \(\newcommand{\lt}{<}\) \(\newcommand{\gt}{>}\) \(\newcommand{\amp}{&}\) \(\definecolor{fillinmathshade}{gray}{0.9}\)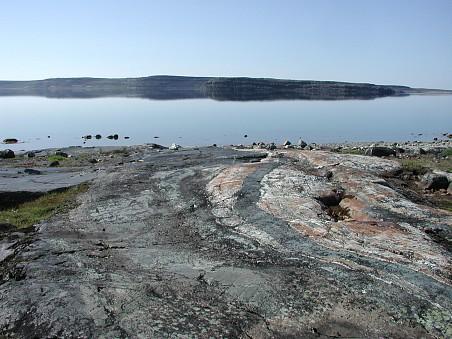
Relative time allows scientists to tell the story of Earth events, but does not provide specific numeric ages, and thus, the rate at which geologic processes operate. Based on Hutton’s principle of uniformitarianism (see Chapter 1), early geologists surmised geological processes work slowly and the Earth is very old. Relative dating principles was how scientists interpreted Earth history until the end of the 19th Century. Because science advances as technology advances, the discovery of radioactivity in the late 1800s provided scientists with a new scientific tool called radioisotopic dating. Using this new technology, they could assign specific time units, in this case years, to mineral grains within a rock. These numerical values are not dependent on comparisons with other rocks such as with relative dating, so this dating method is called absolute dating [5]. There are several types of absolute dating discussed in this section but radioisotopic dating is the most common and therefore is the focus on this section.
Radioactive Decay

All elements on the Periodic Table of Elements (see Chapter 3) contain isotopes. An isotope is an atom of an element with a different number of neutrons. For example, hydrogen (H) always has 1 proton in its nucleus (the atomic number), but the number of neutrons can vary among the isotopes (0, 1, 2). Recall that the number of neutrons added to the atomic number gives the atomic mass. When hydrogen has 1 proton and 0 neutrons it is sometimes called protium (1H), when hydrogen has 1 proton and 1 neutron it is called deuterium (2H), and when hydrogen has 1 proton and 2 neutrons it is called tritium (3H).
Many elements have both stable and unstable isotopes. For the hydrogen example, 1H and 2H are stable, but 3H is unstable. Unstable isotopes, called radioactive isotopes, spontaneously decay over time releasing subatomic particles or energy in a process called radioactive decay. When this occurs, an unstable isotope becomes a more stable isotope of another element. For example, carbon-14 (14C) decays to nitrogen-14 (14N).
The radioactive decay of any individual atom is a completely unpredictable and random event. However, some rock specimens have an enormous number of radioactive isotopes, perhaps trillions of atoms and this large group of radioactive isotopes do have a predictable pattern of radioactive decay. The radioactive decay of half of the radioactive isotopes in this group takes a specific amount of time. The time it takes for half of the atoms in a substance to decay is called the half-life. In other words, the half-life of an isotope is the amount of time it takes for half of a group of unstable isotopes to decay to a stable isotope. The half-life is constant and measurable for a given radioactive isotope, so it can be used to calculate the age of a rock. For example, the half-life uranium-238 (238U) is 4.5 billion years and the half-life of 14C is 5,730 years.
The principles behind this dating method require two key assumptions. First, the mineral grains containing the isotope formed at the same time as the rock, such as minerals in an igneous rock that crystallized from magma. Second, the mineral crystals remain a closed system, meaning they are not subsequently altered by elements moving in or out of them.
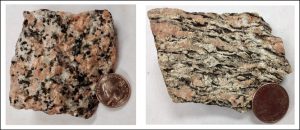
These requirements place some constraints on the kinds of rock suitable for dating, with the igneous rock being the best. Metamorphic rocks are crystalline, but the processes of metamorphism may reset the clock and derived ages may represent a smear of different metamorphic events rather than the age of original crystallization. Detrital sedimentary rocks contain clasts from separate parent rocks from unknown locations and derived ages are thus meaningless. However, sedimentary rocks with precipitated minerals, such as evaporites, may contain elements suitable for radioisotopic dating. Igneous pyroclastic layers and lava flows within a sedimentary sequence can be used to date the sequence.
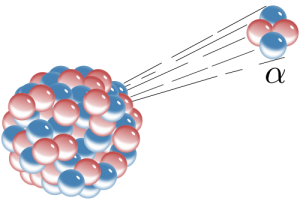
There are several ways radioactive atoms decay. Alpha decay is when an alpha particle, which consists of two protons and two neutrons, is emitted from the nucleus of an atom. The loss of four particles, in this case, two neutrons and two protons, lowers the mass of the atom by four. For example, alpha decay takes place in the unstable isotope 238U, which has an atomic number of 92 (92 protons) and a mass number of 238 (total of all protons and neutrons). When 238U spontaneously emits an alpha particle, it becomes thorium-234 (234Th). The radioactive decay product of an element is called its daughter isotope and the original element is called the parent isotope. In this case, 238U is the parent isotope and 234Th is the daughter isotope. The half-life of 238U is 4.5 billion years, i.e., the time it takes for half of the parent isotope atoms to decay into the daughter isotope. This isotope of uranium, 238U, can be used for absolute dating the oldest materials found on Earth, and even meteorites and materials from the earliest events in our solar system.

It is important to note that 234Th is also not stable. It continues to decay as seen in the figure above until it forms the stable daughter isotope, lead-206 (206Pb). Below is a table of some of the more commonly-used radioactive dating isotopes and their half-lives.
Elements | Parent symbol | Daughter symbol | Half-life |
Uranium-238/Lead-206 | 238U | 206Pb | 4.5 billion years |
Uranium-235/Lead-207 | 235U | 207Pb | 704 million years |
Potassium-40/Argon-40 | 40K | 40Ar | 1.25 billion years |
Rubidium-87/Strontium-87 | 87Rb | 87Sr | 48.8 billion years |
Carbon-14/Nitrogen-14 | 14C | 14N | 5,730 years |
Radioisotopic Dating

For a given sample of rock, how is the dating procedure carried out? The parent and daughter isotopes are separated out of the mineral using chemical extraction. In the case of uranium, 238U and 235U isotopes are separated out together, as are the 206Pb and 207Pb with an instrument called a mass spectrometer [9].
Here is a simple example of age calculation using the daughter-to-parent ratio of isotopes. When the mineral initially forms, it consists of 0% daughter and 100% parent isotope, so the daughter-to-parent ratio (D/P) is 0. After one half-life, half the parent has decayed so there is 50% daughter and 50% parent, a 50/50 ratio, with D/P = 1. After two half-lives, there is 75% daughter and 25% parent (75/25 ratio) and D/P = 3. This can be further calculated for a series of half-lives as shown in the table. The table does not show more than 10 half-lives because, after about 10 half-lives, the amount of remaining parent is so small it becomes too difficult to accurately measure via chemical analysis. Modern applications of this method have achieved remarkable accuracies of plus or minus two million years in 2.5 billion years (that’s ±0.055%) [10]. Applying the uranium/lead technique in any given sample analysis provides two separate clocks running at the same time, 238U and 235U. The existence of these two clocks in the same sample gives a cross-check between the two. Many geological samples contain multiple parent/daughter pairs, so cross-checking the clocks confirms that radioisotopic dating is highly reliable.
Half-lives (#) | Parent present (%) | Daughter present (%) | Daughter/ Parent ratio | Parent/ Daughter ratio |
Start the clock | 100 | 0 | 0 | infinite |
1 | 50 | 50 | 1 | 1 |
2 | 25 | 75 | 3 | 0.33 |
3 | 12.5 | 87.5 | 7 | 0.143 |
4 | 6.25 | 93.75 | 15 | 0.0667 |
5 | 3.125 | 96.875 | 31 | 0.0325 |
10 | 0.098 | 99.9 | 1023 | 0.00098 |
The ratio of parent to a daughter in terms of half-life.
Another radioisotopic dating method involves carbon and is useful for dating archaeologically important samples containing organic substances like wood or bone. Radiocarbon dating, also called carbon dating, uses the unstable isotope carbon-14 (14C). While an organism is alive, the ratio of 14C amounts don’t really change. However, when it dies, the radiocarbon clock starts ticking as the 14C decays back to 14N by beta decay, which has a half-life of 5,730 years. The radiocarbon dating technique is thus useful for 57,300 years or so, about 10 half-lives back. Since rock or sediment were never living, you are not dating the rock or sediment itself but organic material found layered in with the rock or sediment. You can then infer the age of the surrounding material.
Age of the Earth
The work of Hutton and other scientists gained attention after the Renaissance (see Chapter 1), spurring exploration into the idea of an ancient Earth. In the late 19th century William Thompson, a.k.a. Lord Kelvin, applied his knowledge of physics to develop the assumption that the Earth started as a hot molten sphere. He estimated the Earth is 98 million years old, but because of uncertainties in his calculations stated the age as a range of between 20 and 400 million years [12; 13]. Kelvin's numbers were not reliable due to miscalculations related to unequal heat transfer within the Earth. Kelvin failed to consider pliability and convection in the Earth’s mantle as a heat transfer mechanism as well. Kelvin’s estimate for Earth’s age was considered plausible but not without challenge, and the discovery of radioactivity provided a more accurate method for determining ancient ages [14].
In the 1950s, Clair Patterson (1922–1995) thought he could determine the age of the Earth using radioactive isotopes from meteorites, which he considered to be early solar system remnants that were present at the time Earth was forming. Patterson analyzed meteorite samples for uranium and lead using a mass spectrometer. He used the uranium/lead dating technique in determining the age of the Earth to be 4.55 billion years, give or take about 70 million (± 1.5%) [15]. The current estimate for the age of the Earth is 4.54 billion years, give or take 50 million (± 1.1%) [13]. It is remarkable that Patterson, who was still a graduate student at the University of Chicago, came up with a result that has been little altered in over 60 years, even as technology has improved dating methods.
Dating Geological Events
As stated above igneous rock is the best for radioisotopic dating with most elements. Metamorphic rocks are crystalline, but the processes of metamorphism may reset the clock and derived ages may represent a smear of different metamorphic events rather than the age of original crystallization. Detrital sedimentary rocks contain clasts from separate parent rocks from unknown locations and derived ages are thus meaningless. However, sedimentary rocks with precipitated minerals, such as evaporites, may contain elements suitable for radioisotopic dating. Igneous pyroclastic layers and lava flows within a sedimentary sequence can be used to date the sequence.
Sediment that contains carbon may be dated using radiocarbon dating, as long as the sediment is less than about 60,000 years old due to Carbon's short half life discussed above.