6.4.3: Feldspars
- Page ID
- 18870
Feldspars (Ca,Na,K)(Si,Al)4O8 |
|
Most important end members | |
anorthite albite orthoclase |
CaAl2Si2O8 NaAlSi3O8 KAlSi3O8 |
Feldspars are the most abundant minerals in Earth’s crust, in part because they contain six of the seven most abundant elements in the crust. They are widespread and are essential minerals in many igneous, metamorphic, and sedimentary rocks. Feldspars are solid-solution minerals and have the general formula (Ca,Na,K)(Si,Al)4O8. Rarely, they contain significant amounts of other elements such as Ba, Sr, B, or Fe. For most purposes, we consider them to be ternary solutions, which means we can describe their composition in terms of three end members and plot them on diagrams such as the one shown in Figure 6.34 and in Figure 2.22 (Chapter 2). The important feldspar end members are albite (NaAlSi3O8), anorthite (CaAl2Si2O8), and orthoclase (KAlSi3O8).
In Figure 6.34, the blue region shows the compositions of naturally occurring feldspars. Natural feldspars form two distinct series, the alkali feldspar series and plagioclase, both labeled on this triangular diagram. Alkali feldspar, mainly solutions of orthoclase and albite, sometimes contains up to 15 wt % anorthite. Plagioclase, often called plagioclase feldspar, is mostly a solid solution of albite and anorthite, although it may contain up to 10 wt % orthoclase. We call any feldspar with composition near NaAlSi3O8, albite, and one with composition near CaAl2Si2O8, anorthite, even if other components are present. Intermediate plagioclase compositions are commonly given specific names (labeled in the figure): oligoclase, andesine, labradorite, and bytownite. Labradorite, for example, is plagioclase with composition between 70% anorthite-30% albite and 50% anorthite-50% albite. Labradorite may also contain a small amount of orthoclase. Compositions between plagioclase and alkali feldspar (that would plot in the white part of the triangle) are rare or do not exist.
Confusion sometimes arises because the names of some composition ranges are the same as the names of feldspar end members (albite, anorthite, orthoclase). Orthoclase, for example, is the name given to end member KAlSi3O8. It is also the name we give to any feldspar that is >90 KAlSi3O8. The alkali feldspars, with compositions between NaAlSi3O8 and KAlSi3O8 have not been divided into as many bins as plagioclase feldspars. The term anorthoclase, however, is often used to refer to alkali feldspars with intermediate compositions (10-36% KAlSi3O8 with the rest being mostly NaAlSi3O8).
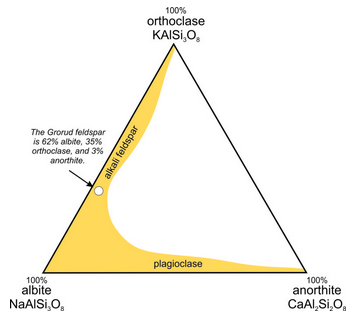
The triangular diagram in Figure 6.35 is similar to the one in Chapter 2 (section 2.5.1); it shows the composition of a feldspar from Grorud, near Oslo, Norway. This feldspar is an example of anorthoclase. It has a composition of about (Ca0.03Na0.62K0.35) (Al1.03Si2.97)O8, so it contains 3 mol % anorthite (CaAl2Si2O8), 62 mol % albite (NaAlSi3O8), and 35 mol % orthoclase (KAlSi3O8). For this feldspar, XAn = 3, XAb = 62, and XOr = 35, where the symbol X stands for mole fraction. Often, we describe the compositions of feldspars by using abbreviations with subscripts. Thus, the Grorud feldspar has composition An3Ab62Or35.
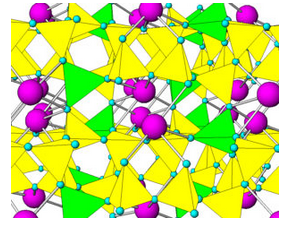
Like quartz, feldspars are framework silicates. Unlike quartz, feldspars contain both SiO4 and AlO4 tetrahedra. As an example, Figure 6.36 shows the atomic arrangement in albite. The purple atoms are sodium, and the yellow and green tetrahedra are (SiO4) and (AlO4), respectively. In all feldspars, Na, K, or Ca occupy spaces between tetrahedra.
Feldspars in Igneous Rocks
Most igneous rocks contain feldspar of some sort, but the kind of feldspar varies with rock composition. In silicic igneous rocks, such as granite, plagioclase is absent or subordinate to K-rich alkali feldspar. If plagioclase is present, it is always albite-rich. Similarly, in mafic rocks, alkali feldspar is not normally present but plagioclase is common. Because mafic rocks contain much more Ca than Na, the plagioclase in them is generally anorthite-rich. Intermediate igneous rocks nearly always contain both feldspars.
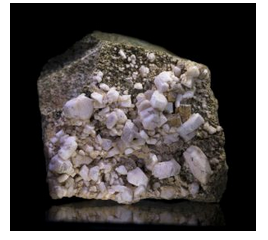
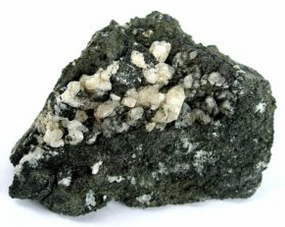
The two photos above show light colored albite crystals from a classic locality in Austria and anorthite crystals from near Naples, Italy. These are both examples of plagioclase.
Feldspars with compositions close to KAlSi3O8 are generally called K-feldspar, or K-spar. But, K-feldspar has three polymorphs with slightly different atomic arrangements: orthoclase, microcline, and sanidine. Photos of each are shown below in Figures 6.39, 6.40, and 6.41. In these photos the polymorphs have different colors, but color is not a good diagnostic property because the different polymorphs all come in many colors. Note the well developed penetration twins in the sanidine specimen.
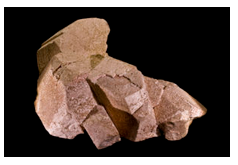
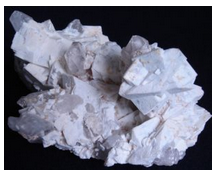
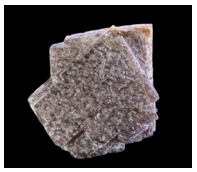
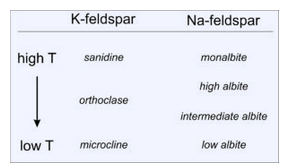
The different K-feldspar polymorphs are stable under different conditions. Sanidine forms in high temperature rocks; orthoclase and microcline form in lower temperature rocks (Figure 6.42). Sanidine commonly crystallizes from silicic lava but may change into orthoclase and, perhaps, microcline if the lava cools relatively slowly. Just about all microcline forms by recrystallization of sanidine or orthoclase.
Like K-feldspar, Na-feldspar forms different polymorphs, with different atomic arrangements, depending on temperature (Figure 6.42). At high temperatures, just below albite’s melting point, we call the feldspar monalbite. Three other polymorphs exist at lower temperatures, all generally called albite. Unlike the SiO2 polymorphs, the differences in atomic structure between the feldspar polymorphs are not great and the boundaries on phase diagrams are poorly known. And, when high-temperature feldspars change into low-temperature ones because of cooling or reequilibration at Earth’s surface, crystal shape may not change even though atoms move and bonds adjust. Distinguishing between the different Na-feldspar polymorphs can be difficult or impossible without X-ray analysis.
Twinning of Feldspars
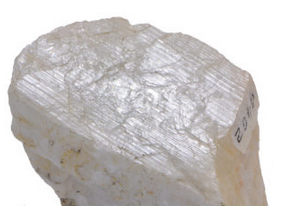
Figure 6.43 shows a plagioclase crystal with twin striations across its top. The striations derive from a type of polysynthetic twinning called albite twinning. The atomic arrangements in alternating domains are slightly different and are reflections of each other. See the drawing of albite twinning in Figure 4.43 (Chapter 4) and look at the photo in Figure 4.44 (Chapter 4) for another example of this kind of twinning.
Twinning occurs in all kinds of feldspars but is generally not visible with the naked eye. So, although a feldspar crystal appears homogeneous, it may be composed of two or more twin domains. We most easily see feldspar twinning when using a polarizing microscope to view a feldspar in a thin section. The different domains are visible because they have different optical properties.
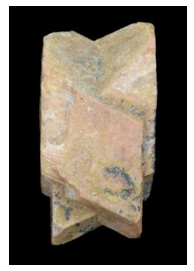
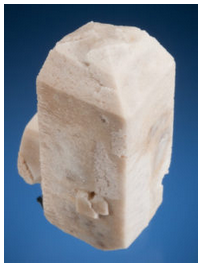
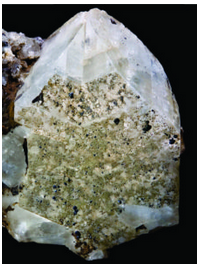
Feldspars twin in several different ways that have different names, for example Carlsbad, Baveno, or Manebach twinning, shown in the three photos above (Figures 6.44, 6.45, and 6.46). The crystals seen in these figures are about 4 cm, 4 cm, and 7 cm tall, respectively. These three kinds of twins are simple twins involving only two twin domains.
Carlsbad twins are penetration twins common in orthoclase, and sometimes in plagioclase. The photo above (left) shows Carlsbad twinning with two orthoclase crystals that appear to have grown through each other. Figures 4.41 (Chapter 4) and 4.42 (Chapter 4) also show Carlsbad twinning. Baveno twins are contact twins that occur most often in orthoclase and microcline. The photo above (center) shows a feldspar with a Baveno twin. A vertical composition plane separates the left and right sides of the crystal. Manebach twins are most common in orthoclase. The photo on the right, above, is a crystal with a Manebach twin. The reentrant angle (two small crystal faces forming a vee shape that points into the crystal) in its top shows where the near-vertical composition plane passes through the crystal. Both Baveno and Manebach twins are rarer than Carlsbad twins. When both are present, Carlsbad and Manebach twinning are diagnostic for orthoclase.
Polysynthetic twins, such as the twins in Figure 6.43 (above), are common in plagioclase and sometimes visible with the naked eye. Albite twinning is one kind; pericline twinning is another. The difference between the two is that domains in albite twins are related by reflection, and domains in pericline twins are related by rotation. Albite twinning is a diagnostic property for plagioclase. Figures 4.43 (Chapter 4)and 4.44 (Chapter 4) show additional examples of albite twinning. Sometimes albite twinning combines with Carlsbad twinning to produce complex crystals, but it is still generally recognizable.
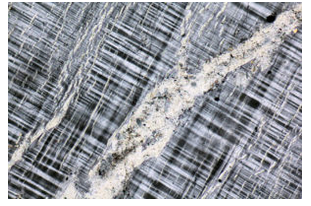
Microcline is the stable K-feldspar polymorph at normal Earth surface conditions. So, other K-feldspars may turn into microcline. Pericline twinning is produced during the polymorphic transformation of sanidine or orthoclase to microcline, and most microcline exhibits both pericline and albite twinning. The combined twinning produces typical microcline twinning, sometimes called cross-hatched twinning or tartan twinning, but we can only see this twinning with a polarizing microscope. Figure 6.47 shows cross-hatched twinning (albite perpendicular to pericline twinning) when viewed with a polarizing microscope. The colors are artifacts and are not the true colors of the domains. The field of view is 2 mm across, and the veins that cut across the field of view contain albite.
Solvus and Miscibility Gaps
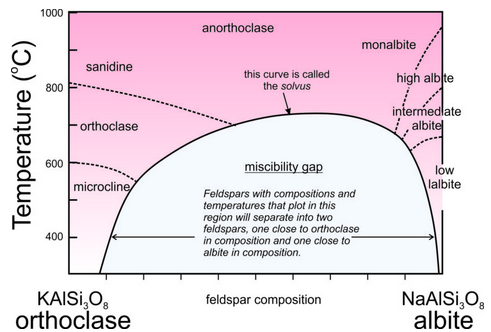
Consider alkali feldspars that are solutions of KAlSi3O8 and NaAlSi3O8. At high temperature, as long as they are not so hot that they melt, these feldspars can have any composition between sanidine (KAlSi3O8) and albite (NaAlSi3O8), and may be anorthoclase (part way between the end members). However, as shown in this phase diagram (Figure 6.48), intermediate compositions (anorthoclase) are stable only at high temperatures. At lower temperatures, instead of having one intermediate feldspar, anorthoclase unmixes to produce two separate feldspars, one K-rich and the other Na-rich. This happens because a miscibility gap exists between albite and orthoclase. A miscibility gap is a composition range within which no single mineral is stable under a particular set of pressure-temperature conditions. It is the gray region shown in the phase diagram. The curve above and around the miscibility gap is called the solvus.
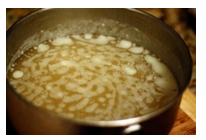
Miscibility gaps are common in mineral systems because some elements do not mix well under all conditions. Many mineral series show complete miscibility at high temperatures, meaning that all compositions are stable. At low temperatures, partial or complete immiscibility restricts possible compositions. We might make an analogy to a pot of homemade chicken soup that separates into two compositions (fat and chicken broth) with cooling (Figure 6.49).
We call the process of a single feldspar separating into two compositions exsolution, equivalent to unmixing. If an intermediate-composition alkali feldspar cools rapidly, it may not have time to exsolve. Thus, we have examples of anorthoclase to study. On the other hand, if cooling is slow, the feldspar will unmix. This may result in separate grains of K-feldspar (orthoclase or microcline) and Na-feldspar (albite) developing in a rock. More often, it results in alternating layers or irregular zones of orthoclase and albite within individual crystals. If the layers or zones are planar or nearly so (appearing long and thin in thin section), we call them exsolution lamellae. The lamellae are sometimes visible with the naked eye, but frequently require a microscope to detect. The figure below (Figure 6.50) shows the surface of a once homogeneous feldspar that has separated into two kinds of lamellae, one K-rich and one Na-rich.
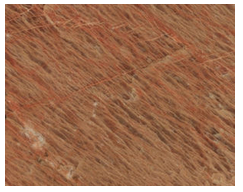
If the original feldspar was K-rich, most of the layers will be K-rich feldspar. After it exsolves, it is technically called perthite. If the original feldspar was Na-rich, most of the layers will be Na-rich, and after exsolution, it is technically called antiperthite. For practical purposes, most mineralogists call any exsolved alkali feldspar perthite, because they need compositional information to distinguish perthite from antiperthite. The photo seen in Figure 6.50 shows perthite with salmon colored veinlets of K-feldspar and lighter colored veinlets of Na-feldspar. This is a polished slab of rock; the view is 4.5 cm across.
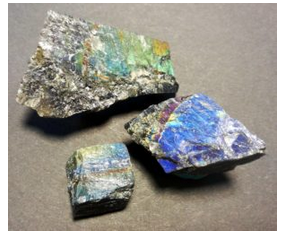
Because they exsolve, alkali feldspars form what is called a limited solid solution at lower temperatures. Intermediate compositions between orthoclase and albite do not exist. In contrast, intermediate plagioclase between albite and anorthite is common. At high temperatures all compositions are stable and, with cooling, there is generally no significant exsolution. But, several small solvi exist at very low temperature which – if cooling is slow enough – can result in microscopic unmixing. The exsolution may give the feldspars an iridescence or a play of colors that helps to identify them. Labradorite, for example, is identified by its labradorescence, a bluish schiller, caused by exsolution. Figure 6.51 show several examples of labradorite; see also Figure 3.53 (Chapter 3). Because exsolution, if present in plagioclase, is on a very fine scale, mineral properties are relatively homogeneous. So, for most purposes, we can ignore the presence or absence of exsolution in plagioclase.
Miscibility Gaps
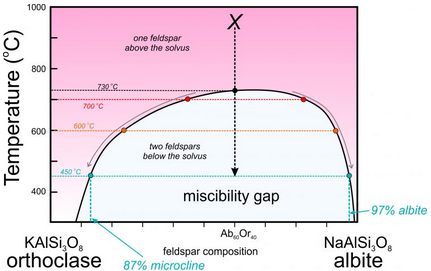
This figure (6.52) is a slight modification of the temperature-composition (T-X) phase diagram in Figure 6.48.
Consider a feldspar of composition Ab60Or40 (marked with an X on the diagram). At high temperature, it will exist as one alkali feldspar (anorthoclase). As it cools to low temperature it separates into two feldspars beginning at about 730 oC. At 700 oC, for example, the compositions of the two feldspars are shown as red dots. At 600 oC, the two compositions are shown as orange dots. And at 450 oC, the compositions are shown by light blue dots. The compositions start at Ab60Or40 and then follow the solvus toward orthoclase and albite (gray arrows) as temperature decreases. At 450 oC, the composition of the albite-rich feldspar will be 97% NaAlSi3O8, while that of the orthoclase-rich feldspar will be about Ab13Or87. The K-feldspar polymorph stable at low temperature is microcline. So, if the feldspar cools completely, the final result will be a mix of microcline-rich feldspar and nearly pure albite, and because the overall composition is closer to albite than orthoclase, there will be more albite than microcline. The likely result will be anitperthite – a single feldspar crystal that contains exsolution lamellae of different compositions
Exsolution and Ternary Feldspars
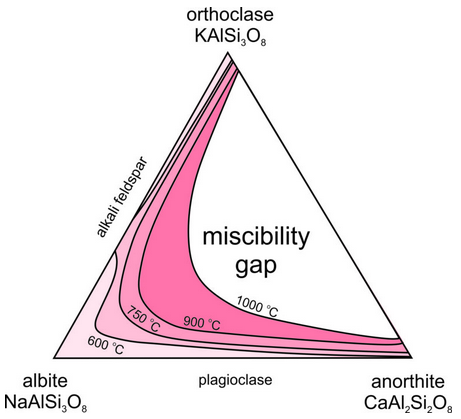
Above, we considered exsolution in alkali feldspars, K-Na feldspars. But, what about ternary feldspars, K-Na-Ca feldspars? As noted, both alkali feldspar and plagioclase exist in a wide range of igneous rocks. But, feldspars with compositions in the middle of the feldspar ternary (shown in white in Figure 6.53) are rarely found because they unmix to form coexisting alkali feldspar and plagioclase. There is a large miscibility gap in the ternary system. At 1000 oC all feldspar compositions, except those that plot in the white region, are stable. But, as temperature decreases the miscibility gap grows wider (show by the temperature contour lines), and if temperature gets as low as 600 oC, feldspar is limited to compositions shown in the lightest pink in this figure.
Feldspar Crystallization – Plagioclase
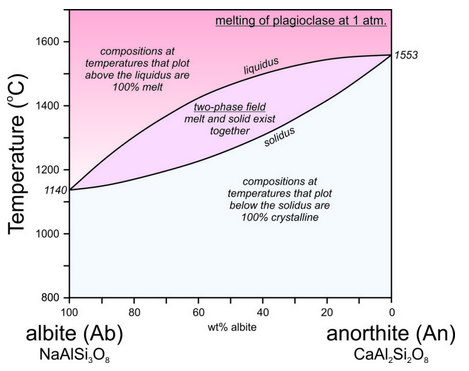
The crystallization temperatures of the two plagioclase end members are different. Albite crystallizes at 1140 oC and anorthite crystallizes at 1553 oC (Figure 6.54). For compositions between albite and anorthite, crystallization is complicated (see Box 6-3) because when an intermediate feldspar begins to crystallize, the first crystal produced is more calcium-rich than the original melt itself. In effect, the anorthite part of the feldspar crystallizes faster than the albite part. If an intermediate plagioclase is partially crystallized, the melt will become more albite-rich. The feldspar that crystallized will therefore have to be more anorthite-rich than the original melt. So, end-member albite and anorthite crystallize congruently. This means they crystallize at specific temperatures and the crystals are the same composition as the melt. But, intermediate composition feldspars crystallize incongruently. They crystallize over a range of temperature and the crystal produced is not the same composition as the melt until crystallization is complete. The temperature at which crystallization begins for a specific composition feldspar is shown by the liquidus curve in the diagram above. Crystallization starts and then continues until temperature reaches the solidus. At temperatures between the liquidus and solidus, in the two-phase field, crystals and melt exist together and are of different compositions.
The Plagioclase Phase Diagram and Fractional Crystallization
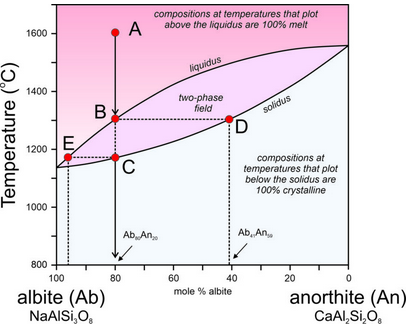
Figure 6.55 is modified from Figure 6.54, the T-X phase diagram describing plagioclase melting and crystallization at 1 atm pressure.
At the highest temperatures, above the liquidus, plagioclase of any composition will be melted. At the lowest temperatures, below the solidus, any plagioclase composition will be entirely crystalline. In between, in the two-phase field, solid crystals and melt will coexist but be of different compositions. This is best described by example.
Point A represents an albite-rich feldspar melt at 1,600 °C. The melt has the same composition as plagioclase that is 80% albite and 20% anorthite (Ab80An20) but is completely molten. As the melt cools, at about 1,300 °C, the first crystals begin to form (point B). A horizontal line at 1,300 °C intersects the liquidus at the melt composition (Point B) and the solidus at the initial crystal composition (Point D). Thus, when crystallization begins, the liquid still has its original composition, but the crystals that start to form are considerably more anorthite-rich than the melt. They have composition Ab41An59.
As the melt continues cooling, crystals continue to form, but they change composition from D to C, following the solidus as temperature decreases. Previously crystallized plagioclase reacts with the melt, so crystal composition changes with cooling and all crystals are homogeneous and have the same composition. A horizontal line can be drawn at any temperature. The intersection of the line with the solidus indicates plagioclase composition and the intersection of the line with the liquidus indicates the composition of the remaining melt. Because the crystals are more anorthite-rich than the melt, the melt becomes more albite-rich as crystallization continues.
As crystallization goes to completion, plagioclase crystals change in composition from Ab41An59 (Point D) to Ab80An20 (the composition of the original melt). Simultaneously, melt composition changes from Ab80An20 to an extremely albite-rich composition (Ab96An04), indicated by Point E on the diagram. At about 1,165 °C, the last drop of melt will crystallize. And, if everything stayed in equilibrium, after complete crystallization, all plagioclase crystals would have the same composition, which must equal that of the original melt.
So, if the crystallization process always went to completion, plagioclase crystals would always end up the same composition as the original melt. However, several things can disrupt this equilibrium. Often crystallization occurs so fast that crystals do not have time to react with the melt as they form. In such cases, the first plagioclase to crystallize is preserved in the centers of large crystals. The crystals are compositionally zoned, the outer zones being more albite-rich. Another complication arises if crystals and melt get separated during the crystallization process. This may occur due to crystal settling, or because remaining melt “squirts off” after the melt is partially solidified. In such cases, crystal-melt equilibrium is not maintained. Both processes are kinds of fractional crystallization that can create melts of different compositions from their parent melts.
Feldspar Crystallization – K-feldspar
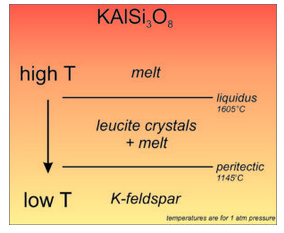
K-rich feldspars can crystallize from a magma, but they exhibit an interesting kind of incongruent crystallization. Consider a melt with composition KAlSi3O8. If it cools, it will eventually reach the liquidus temperature and crystallization will begin. But, as shown here (Figure 6.56), the initial crystals formed will be leucite (KAlSi2O6) instead of K-feldspar (KAlSi3O8). So, as crystallization proceeds, the remaining melt will become more SiO2-rich. Finally, when the temperature reaches what is called the peritectic temperature, the leucite crystals will react with the remaining melt to produce K-feldspar and all will be solid. The difference between liquidus and peritectic temperatures is about 400 °C depending on pressure. If everything stays in equilibrium, we can ignore the temporary presence of leucite crystals. But, sometimes liquid and crystal become separated and leucite may be left behind.
Feldspar Crystallization – Alkali Feldspars
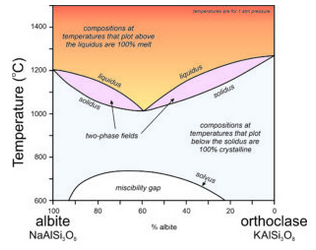
The discussion above is directed at crystallization of K-feldspar, but most alkali feldspars are solid solutions between orthoclase and albite. They are (K,Na)AlSi3O8 feldspars. This complicates crystallization relationships considerably. The phase diagram in Figure 6.57 ignores the incongruent crystallization that would complicate the left side at high temperatures. Assuming that leucite does not crystallize, or that we can ignore it, the alkali feldspar series involves a pair of two-phase fields, similar to the two-phase field on the plagioclase phase diagram (Figure 6.54). There is a temperature minimum (at about 1000 ̊C) for a composition of about Ab60Or40 – this is the lowest temperature where melt can exist. All other compositions are completely crystallized at this temperature.
If melt and crystals stay in equilibrium, when the temperature drops below 1000 ̊C, any composition alkali feldspar will be entirely crystalline and will have the same composition as the original melt. However, the solvus and miscibility gap at lower temperature mean that with more cooling, intermediate composition feldspars will exsolve. They will separate into an albite-rich feldspar and an orthoclase-rich feldspar. The likely result is that perthite will form.