20.2: Evidence of glaciation
- Page ID
- 22759
\( \newcommand{\vecs}[1]{\overset { \scriptstyle \rightharpoonup} {\mathbf{#1}} } \)
\( \newcommand{\vecd}[1]{\overset{-\!-\!\rightharpoonup}{\vphantom{a}\smash {#1}}} \)
\( \newcommand{\id}{\mathrm{id}}\) \( \newcommand{\Span}{\mathrm{span}}\)
( \newcommand{\kernel}{\mathrm{null}\,}\) \( \newcommand{\range}{\mathrm{range}\,}\)
\( \newcommand{\RealPart}{\mathrm{Re}}\) \( \newcommand{\ImaginaryPart}{\mathrm{Im}}\)
\( \newcommand{\Argument}{\mathrm{Arg}}\) \( \newcommand{\norm}[1]{\| #1 \|}\)
\( \newcommand{\inner}[2]{\langle #1, #2 \rangle}\)
\( \newcommand{\Span}{\mathrm{span}}\)
\( \newcommand{\id}{\mathrm{id}}\)
\( \newcommand{\Span}{\mathrm{span}}\)
\( \newcommand{\kernel}{\mathrm{null}\,}\)
\( \newcommand{\range}{\mathrm{range}\,}\)
\( \newcommand{\RealPart}{\mathrm{Re}}\)
\( \newcommand{\ImaginaryPart}{\mathrm{Im}}\)
\( \newcommand{\Argument}{\mathrm{Arg}}\)
\( \newcommand{\norm}[1]{\| #1 \|}\)
\( \newcommand{\inner}[2]{\langle #1, #2 \rangle}\)
\( \newcommand{\Span}{\mathrm{span}}\) \( \newcommand{\AA}{\unicode[.8,0]{x212B}}\)
\( \newcommand{\vectorA}[1]{\vec{#1}} % arrow\)
\( \newcommand{\vectorAt}[1]{\vec{\text{#1}}} % arrow\)
\( \newcommand{\vectorB}[1]{\overset { \scriptstyle \rightharpoonup} {\mathbf{#1}} } \)
\( \newcommand{\vectorC}[1]{\textbf{#1}} \)
\( \newcommand{\vectorD}[1]{\overrightarrow{#1}} \)
\( \newcommand{\vectorDt}[1]{\overrightarrow{\text{#1}}} \)
\( \newcommand{\vectE}[1]{\overset{-\!-\!\rightharpoonup}{\vphantom{a}\smash{\mathbf {#1}}}} \)
\( \newcommand{\vecs}[1]{\overset { \scriptstyle \rightharpoonup} {\mathbf{#1}} } \)
\( \newcommand{\vecd}[1]{\overset{-\!-\!\rightharpoonup}{\vphantom{a}\smash {#1}}} \)
Why do geologists think there were ice ages during the Neoproterozoic? These cold times in Earth history are times when glaciers grew, flowed, and moved sediment around. Though the ice itself melts (and thus cannot be preserved though geologic time), its impacts on the sedimentary record are distinctive and durable. Ancient glaciers left behind three kinds of signatures: (1) striations, (2) tillites, and (3) dropstones. These signatures are found in Neoproterozoic strata in many places: for the Sturtian, there are 39 localities documented, on six continents. For the Marinoan glaciation, there are 48 places where glaciogenic signatures have been found.
Striations
Striations are scratches or grooves that result when a glacier takes a particle of rock (like a cobble or boulder) and drags it against the bedrock, gouging out a scratch mark that shows the direction the glacier was flowing. These are subtle features in cross-section, and they show almost no volume, so the best way to see them is on the ancient bedrock surface over which the glacier flowed. But this is rarely exposed, so this is the least common kind of evidence for Snowball Earth.
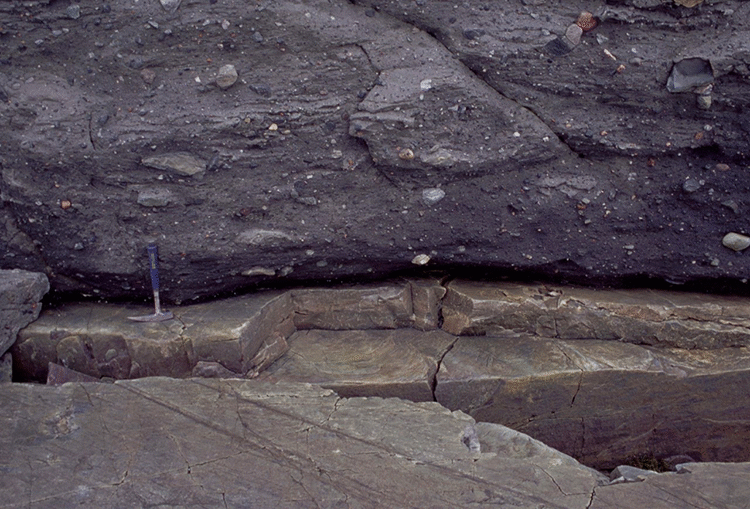
Tillites & diamictites

Glaciers produce deposits of exceptionally poorly sorted sediment: giant particles like boulders are dumped in the same spot as very fine particles like silt and clay. On the continents, this distinctive “boulder clay” is officially called “till.” Similar extremely poorly sorted deposits occur in the marine realm, where glaciers flow into the sea. To get a sense of this style of sorting, and a general sense of the diversity of rock types making up large clasts, as well as the shape of these clasts, we have compiled some representative imagery here.
Some of these examples are modern, and some are ancient. Regardless, they all share key characteristics worth noting.
Check out this gigapixel panorama of Pleistocene-aged till from Alberta, Canada:
(Callan Bentley GigaPan)
You’ll notice there that (1) it’s really poorly sorted, (2) there’s a lot of small stuff —the “matrix” — which “supports” the overall deposit and that means that (3) the biggest bits — the “outsized clasts” — are pretty much isolated from one another, making them look like they are “floating” in the soup of the surrounding clay and sand.
Here’s a 3D model, showing a similar looking deposit from Ireland:
(Callan Bentley & Sadhbh Baxter 3D model)

Again, this is Pleistocene rather than Neoproterozoic, but the darned-near-present is the key to the past. Note the large faceted clasts “floating” (i.e., not touching each other) in all orientations within the finer-grained matrix. When till is lithified (turned to rock through compression and cementation), we call it tillite. The Snowball Earth glaciations are recorded by tillite deposits.
Another rock name frequently used in describing poorly sorted sedimentary deposits is diamictite. Diamictites are poorly sorted, but the name is purely descriptive, and doesn’t necessarily imply a glacial origin for the deposit. For instance, a landslide could produce a very poorly sorted mix of sedimentary grains, a diamicton, which might then be lithified to make a diamictite. Both rock types contain large “out-sized” clasts (cobbles, boulders) is isolated from one another, surrounded by finer-grained, poorly sorted sediment. All tillites are diamictites, but not all diamictites are tillites.







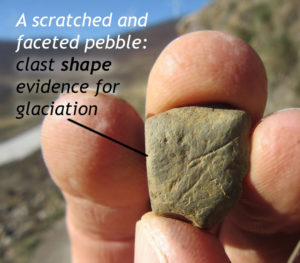
One way to distinguish whether a diamictite had a glacial origin (that is, whether it is a tillite) is on the basis of the character of the larger clasts. Some of the boulders and cobbles in a true tillite will show faceted shapes, where they’ve have flat faces ground down by being dragged along over resistant surfaces. These facets will frequently show randomly-oriented scratches, too. These features together provide compelling evidence of the past grinding action of glacial ice.
Because scratched-up clasts are relatively uncommon, many geologists will be cautious and conservative when describing poorly sorted sedimentary deposits, referring to them by the broader and less specific “diamictite” even if they suspect the deposit is glacial in origin (glaciogenic; that is, a tillite or its marine equivalent).
Here are two hand samples of diamictite to examine. Which one is clearly glaciogenic? Which one is equivocal? Challenge yourself to explain the evidence you’re keying into to make the distinction.
(Callan Bentley GIGAmacro)
(Robin Rohrback GIGAmacro)
Metadiamictites

Of course, like any rock, diamictites are subject to metamorphism if mountain-building occurs in their region after they form. Metamorphism transforms diamictites into metadiamictites, and tillites into metatillites. Metadiamictites show the original sedimentary poor sorting, but then also bear a foliated metamorphic overprint, giving them a palimpsest texture that indicates this more complicated history.
These are potentially-glacial deposits that were deposited in regions that later experienced mountain-building and recrystallization. If the regional metamorphism is intense, original primary sedimentary structures can be destroyed. If metamorphism is moderate, it can generate confusion, as metamorphic foliation wraps around outsized clasts in the same way that sedimentary laminations might in an unmetamorphosed dropstone-bearing deposit. Geologists working in metamorphic terranes have to be extra careful in making sedimentary interpretations of such rocks.
Compare the two formation mechanisms here:
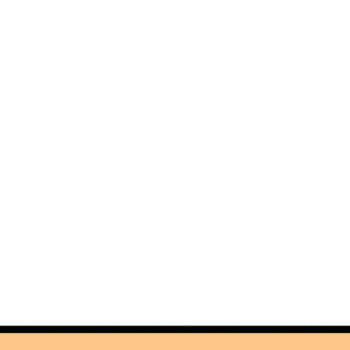

Here is a gallery of metadiamictites to peruse:

















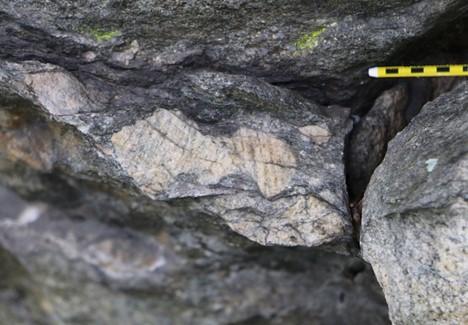

Did I Get It? - Quiz
Is this a non-glaciogenic diamictite or a tillite (with a glacial origin)?
a. It's a tillite, because this large clast shows scratches on at least one of its faceted surfaces.
b. It's a tillite, because this large clast is pink.
c. All we can say is that it is a diamictite, since the rock is poorly sorted.
d. All we can say is that it is a diamictite, since there is no distinctive glaciogenic evidence here.
- Answer
-
a. It's a tillite, because this large clast shows scratches on at least one of its faceted surfaces.
Summarize the steps in the making of a glaciogenic metadiamictite (a metatillite) in chronological order.
a.
1) Glaciation and erosion of pre-existing rocks
2) Metamorphism of the diamictite (tillite)
3) Lithification of the sediment into diamictite (tillite)
4) Deposition of poorly sorted sediment
b.
1) Glaciation and erosion of pre-existing rocks
2) Deposition of poorly sorted sediment
3) Lithification of the sediment into diamictite (tillite)
4) Metamorphism of the diamictite (tillite)
c.
1) Intrusion of granite
2) Metamorphism of the granite
3) Uplift and erosion of the meta-granite
4) Glaciation
- Answer
-
b.
1) Glaciation and erosion of pre-existing rocks
2) Deposition of poorly sorted sediment
3) Lithification of the sediment into diamictite (tillite)
4) Metamorphism of the diamictite (tillite
Is this a tillite? Explain.
a. No, this is a limestone with mud-chip rip-up clasts.
b. No, it's better sorted than a tillite (the pebbles are touching, not "floating"), and there is no sign of scratching on the clasts.
c. Yes, this sample shows an extremely poorly sorted mixture of large "out-sized" cobbles that "float" in a matrix of much finer sediment. Several of the big clasts show scratches on their faceted surfaces.
d. Yes, it's dark colored and shows a mixture of rounded and semiangular clasts.
- Answer
-
b. No, it's better sorted than a tillite (the pebbles are touching, not "floating"), and there is no sign of scratching on the clasts.
Dropstones
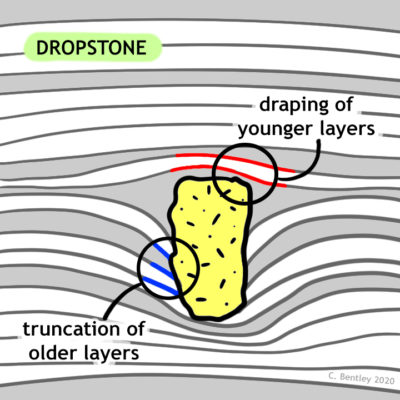
A third and final signature of glaciation in the Neoproterozoic era is the presence of dropstones in offshore marine sedimentary deposits. These are fine-grained, thin-bedded or laminated deepwater deposits of clay and silt, into which drop much larger particles, such as pebbles, cobbles, or boulders. The dropstones pierce into the soft pre-existing sedimentary layers, disrupting their continuity. We say that the pre-dropstone layers are truncated. Post-dropstone layers of fine sediment are draped atop them.
There’s more than one way to make dropstones today. After all, you could paddle a canoe out to deep water with a few cobbles onboard, then toss them in with a splash. Or a tree could grow through many years, its roots wrapping around cobbles in the soil. If the tree were to be blown over in a storm, it might be washed out to sea, root-wrapped cobbles and all. When, at sea, the wood finally rots, the cobble would be freed to drop into deep water sediments.
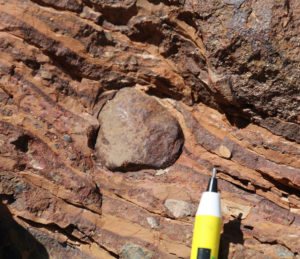
But there were neither trees nor canoes during the Neoproterozoic. The only plausible candidate for transporting diverse clasts offshore where they could be dropped into thin sedimentary layers was ice. The idea is that when outlet glaciers from the Snowball Earth ice sheets reached the sea, where they calved off icebergs full of gravel, sand, cobbles, and silt. These icebergs floated out to deep water, melted, and let their load of sediment fall through the water below.
[Cartoon of larger-scale process here? Simplified version of this: https://www.nature.com/articles/s41467-019-13496-5/figures/2]
In the Neoproterozoic, ice was the only way to get dropstones, and so the presence of Neoproterozoic dropstones = Neoproterozoic glacial ice.
(Robin Rohrback GIGAmacro)
It’s worth pointing out that there’s no size implication with the term “dropstone.” Dropstones can be huge, but they can also be small. Here’s a very small example: a mere 40 microns across, imaged with a scanning electron microscope:
(Robin Rohrback SEM GIGAmacro)
Here is a gallery of dropstones to peruse:












Did I Get It? - Quiz
What feature is shown in this sample?
a. A dropstone in a diamictite
b. Striations on a glacial pavement
c. Cap carbonate
- Answer
-
a. A dropstone in a diamictite
What characterizes a tillite, as opposed to a diamictite?
a. Diamictites are lithified, but tillites are not.
b. Diamictites are poorly sorted sedimentary deposits that are lithified, but tillites are diamictites that are specifically glacial in origin.
c. Diamictites are well sorted, but tillites are poorly sorted.
d. Tillites are well sorted, but diamictites are poorly sorted.
- Answer
-
b. Diamictites are poorly sorted sedimentary deposits that are lithified, but tillites are diamictites that are specifically glacial in origin.
Relative to a tillite, we might look for striations _________________.
a. ...on top of the tillite, on the surface separating it from overlying "cap carbonate" deposits.
b. ...below the tillite, on the unconformity surface separating it from the bedrock beneath.
c. ...within the tillite, between any dropstones.
- Answer
-
b. ...below the tillite, on the unconformity surface separating it from the bedrock beneath.