11.2: Materials Produced by Volcanic Eruptions
- Page ID
- 20166
\( \newcommand{\vecs}[1]{\overset { \scriptstyle \rightharpoonup} {\mathbf{#1}} } \)
\( \newcommand{\vecd}[1]{\overset{-\!-\!\rightharpoonup}{\vphantom{a}\smash {#1}}} \)
\( \newcommand{\id}{\mathrm{id}}\) \( \newcommand{\Span}{\mathrm{span}}\)
( \newcommand{\kernel}{\mathrm{null}\,}\) \( \newcommand{\range}{\mathrm{range}\,}\)
\( \newcommand{\RealPart}{\mathrm{Re}}\) \( \newcommand{\ImaginaryPart}{\mathrm{Im}}\)
\( \newcommand{\Argument}{\mathrm{Arg}}\) \( \newcommand{\norm}[1]{\| #1 \|}\)
\( \newcommand{\inner}[2]{\langle #1, #2 \rangle}\)
\( \newcommand{\Span}{\mathrm{span}}\)
\( \newcommand{\id}{\mathrm{id}}\)
\( \newcommand{\Span}{\mathrm{span}}\)
\( \newcommand{\kernel}{\mathrm{null}\,}\)
\( \newcommand{\range}{\mathrm{range}\,}\)
\( \newcommand{\RealPart}{\mathrm{Re}}\)
\( \newcommand{\ImaginaryPart}{\mathrm{Im}}\)
\( \newcommand{\Argument}{\mathrm{Arg}}\)
\( \newcommand{\norm}[1]{\| #1 \|}\)
\( \newcommand{\inner}[2]{\langle #1, #2 \rangle}\)
\( \newcommand{\Span}{\mathrm{span}}\) \( \newcommand{\AA}{\unicode[.8,0]{x212B}}\)
\( \newcommand{\vectorA}[1]{\vec{#1}} % arrow\)
\( \newcommand{\vectorAt}[1]{\vec{\text{#1}}} % arrow\)
\( \newcommand{\vectorB}[1]{\overset { \scriptstyle \rightharpoonup} {\mathbf{#1}} } \)
\( \newcommand{\vectorC}[1]{\textbf{#1}} \)
\( \newcommand{\vectorD}[1]{\overrightarrow{#1}} \)
\( \newcommand{\vectorDt}[1]{\overrightarrow{\text{#1}}} \)
\( \newcommand{\vectE}[1]{\overset{-\!-\!\rightharpoonup}{\vphantom{a}\smash{\mathbf {#1}}}} \)
\( \newcommand{\vecs}[1]{\overset { \scriptstyle \rightharpoonup} {\mathbf{#1}} } \)
\( \newcommand{\vecd}[1]{\overset{-\!-\!\rightharpoonup}{\vphantom{a}\smash {#1}}} \)
\(\newcommand{\avec}{\mathbf a}\) \(\newcommand{\bvec}{\mathbf b}\) \(\newcommand{\cvec}{\mathbf c}\) \(\newcommand{\dvec}{\mathbf d}\) \(\newcommand{\dtil}{\widetilde{\mathbf d}}\) \(\newcommand{\evec}{\mathbf e}\) \(\newcommand{\fvec}{\mathbf f}\) \(\newcommand{\nvec}{\mathbf n}\) \(\newcommand{\pvec}{\mathbf p}\) \(\newcommand{\qvec}{\mathbf q}\) \(\newcommand{\svec}{\mathbf s}\) \(\newcommand{\tvec}{\mathbf t}\) \(\newcommand{\uvec}{\mathbf u}\) \(\newcommand{\vvec}{\mathbf v}\) \(\newcommand{\wvec}{\mathbf w}\) \(\newcommand{\xvec}{\mathbf x}\) \(\newcommand{\yvec}{\mathbf y}\) \(\newcommand{\zvec}{\mathbf z}\) \(\newcommand{\rvec}{\mathbf r}\) \(\newcommand{\mvec}{\mathbf m}\) \(\newcommand{\zerovec}{\mathbf 0}\) \(\newcommand{\onevec}{\mathbf 1}\) \(\newcommand{\real}{\mathbb R}\) \(\newcommand{\twovec}[2]{\left[\begin{array}{r}#1 \\ #2 \end{array}\right]}\) \(\newcommand{\ctwovec}[2]{\left[\begin{array}{c}#1 \\ #2 \end{array}\right]}\) \(\newcommand{\threevec}[3]{\left[\begin{array}{r}#1 \\ #2 \\ #3 \end{array}\right]}\) \(\newcommand{\cthreevec}[3]{\left[\begin{array}{c}#1 \\ #2 \\ #3 \end{array}\right]}\) \(\newcommand{\fourvec}[4]{\left[\begin{array}{r}#1 \\ #2 \\ #3 \\ #4 \end{array}\right]}\) \(\newcommand{\cfourvec}[4]{\left[\begin{array}{c}#1 \\ #2 \\ #3 \\ #4 \end{array}\right]}\) \(\newcommand{\fivevec}[5]{\left[\begin{array}{r}#1 \\ #2 \\ #3 \\ #4 \\ #5 \\ \end{array}\right]}\) \(\newcommand{\cfivevec}[5]{\left[\begin{array}{c}#1 \\ #2 \\ #3 \\ #4 \\ #5 \\ \end{array}\right]}\) \(\newcommand{\mattwo}[4]{\left[\begin{array}{rr}#1 \amp #2 \\ #3 \amp #4 \\ \end{array}\right]}\) \(\newcommand{\laspan}[1]{\text{Span}\{#1\}}\) \(\newcommand{\bcal}{\cal B}\) \(\newcommand{\ccal}{\cal C}\) \(\newcommand{\scal}{\cal S}\) \(\newcommand{\wcal}{\cal W}\) \(\newcommand{\ecal}{\cal E}\) \(\newcommand{\coords}[2]{\left\{#1\right\}_{#2}}\) \(\newcommand{\gray}[1]{\color{gray}{#1}}\) \(\newcommand{\lgray}[1]{\color{lightgray}{#1}}\) \(\newcommand{\rank}{\operatorname{rank}}\) \(\newcommand{\row}{\text{Row}}\) \(\newcommand{\col}{\text{Col}}\) \(\renewcommand{\row}{\text{Row}}\) \(\newcommand{\nul}{\text{Nul}}\) \(\newcommand{\var}{\text{Var}}\) \(\newcommand{\corr}{\text{corr}}\) \(\newcommand{\len}[1]{\left|#1\right|}\) \(\newcommand{\bbar}{\overline{\bvec}}\) \(\newcommand{\bhat}{\widehat{\bvec}}\) \(\newcommand{\bperp}{\bvec^\perp}\) \(\newcommand{\xhat}{\widehat{\xvec}}\) \(\newcommand{\vhat}{\widehat{\vvec}}\) \(\newcommand{\uhat}{\widehat{\uvec}}\) \(\newcommand{\what}{\widehat{\wvec}}\) \(\newcommand{\Sighat}{\widehat{\Sigma}}\) \(\newcommand{\lt}{<}\) \(\newcommand{\gt}{>}\) \(\newcommand{\amp}{&}\) \(\definecolor{fillinmathshade}{gray}{0.9}\)Volcanic eruptions produce three types of materials: gas, lava, and fragmented debris called tephra.
Volcanic Gas
Magma contains gas. Most of the gas emitted by volcanoes is water, but gas also contains carbon dioxide (CO2), sulphur dioxide (SO2), and hydrogen sulphide (H2S), in order of decreasing abundance.
.At high pressures, the gases are dissolved in the magma, but if the pressure decreases, the gas comes out of solution, forming bubbles, similar to what happens when you open a bottle of pop. Pop is bottled under pressure, forcing carbon dioxide gas to dissolve into the fluid. As long as the bottle is closed and the pressure remains high, you’ll see few to no bubbles in the pop. But if you open the bottle, air rushes out, decreasing the pressure on the pop. The pop will begin to fizz as carbon dioxide gas comes out of solution and forms bubbles.
Volcanoes release gases when erupt, and through openings called fumaroles (Figure 11.7). They can also release gas into soil and groundwater.

Lava
The ease with which lava flows, and the structures it forms depend on how much silica and gas are in the lava. The more silica, the more polymerization (formation of long molecules) happens, stiffening the lava. The stiffness of lava is described in terms of viscosity: lava that flows easily has low viscosity, and lava that is sticky and stiff has high viscosity.
In general, higher-silica (felsic) lava contains more gas than low-silica lava. When the gas forms into bubbles, viscosity increases further. Consider the pop analogy again: If you shook the bottle vigorously, then opened it, the pop would gush out in a thick, frothy flow. In contrast, if you took care to not shake the bottle before opening it, you could pour out a thin stream of fluid. The presence of gas not only makes high-silica lava more viscous, but can affect mafic lavas in that way as well.
Concept Check: Viscosity
Query \(\PageIndex{1}\)
The thickness and shape of a lava flow depends on its viscosity. The greater the viscosity, the thicker the flow, and the shorter the distance it can go before solidifying. Highly viscous lava might not flow very far at all, and simply accumulate as a bulge, called a lava dome, in a volcano’s crater. Figure 11.8 shows a dome formed from rhyolitic lava in the crater of Mt. St. Helens.

Less viscous andesitic lava can travel further, as with the thick flow in Figure 11.9 (right). The left of Figure 11.9 shows thin streams of freely-flowing, low-silica, low-viscosity basaltic lava.

Low-viscosity basaltic lava flows may travel extended distances if they move through conduits called lava tubes. These are tunnels within older solidified lava flows. Figure 11.10 (top) shows a view into a lava tube through a hole in the overlying rock, called a skylight. Figure 11.10 (bottom) shows the interior of a lava tube, with a person for scale. Lava tubes form naturally and readily because flowing mafic lava preferentially cools near its margins, forming solid lava levées that eventually close over the top of the flow. Lava within tubes can flow for 10s of km because the tubes insulate the lava from the atmosphere and slow the rate at which the lava cools. The Hawai’ian volcanoes are riddled with thousands of old, drained lava tubes, some as long as 50 km.

Lava Structures
Pahoehoe
Lava flowing on the surface can take on different shapes as it cools. Basaltic lava with an unfragmented surface (e.g., Figure 11.9, right), is called pahoehoe. (pronounced pa-hoy-hoy). Pahoehoe can be smooth and billowy. It can also develop a wrinkled texture, called ropy lava (Figure 11.11). Ropy lava forms when the outermost layer of the lava cools and develops a skin (visible as a dark layer in Figure 11.11, left), but the skin is still hot and thin enough to be flexible. The skin is stiffer than the lava beneath it, and is dragged by flowing lava and folded up into wrinkles. Figure 11.11 (right) is a close-up view after a cut has been made to show the internal structure of a wrinkled lava flow. Notice the many holes, or vesicles, within the lava, formed when the lava solidified around gas bubbles.

A’a and Blocky Lava
When the outer layer of the lava flow can’t accommodate the motion of lava beneath by deforming smoothly, the outer layer will break into fragments as lava moves beneath it. This could happen if the lava flow develops a thicker, more brittle outer layer, or if it moves faster. The result is a sharp and splintery rubble-like lava flow called a’a (pronounced like “lava” but without the l and v). Figure 11.12 (left) shows a close-up view of the advancing front of an a’a lava flow (the flow is moving toward the viewer). Figure 11.12 (right) shows an a’a lava flow viewed from the side. Compare the texture of the a’a flow with the texture of the lighter-grey pahoehoe lava in the foreground of the picture.

Higher viscosity andesitic lava flows also develop a fragmented surface, called blocky lava. This is visible in the toe of the andesitic lava flow from Figure 11.9 (right). The difference between a’a and the andesitic blocky lava is that the blocky lava has fragments with smoother surfaces and fewer vesicles.
Lava Pillows
When lava flows into water, the outside of the lava cools quickly, making a tube (Figure 11.13 (top left)). Blobs of lava develop at the end of the tube (Figure 11.13 (top right)), forming pillows. The bottom left of Figure 10.13 shows pillows covering the sea floor, and the bottom right shows the distinctive rounded shape of pillows in outcrop. Because pillows always form underwater, finding them in the rock record gives us information that the environment was underwater.

Columnar Joints
When lava flows cool and solidify, they shrink. Long vertical cracks, or joints, form within the brittle rock to allow for the shrinkage. Viewed from above, the joints form polygons with 5, 6, or 7- sides, and angles of approximately 120º between sides (Figure 11.14).

Figure 11.15 shows a side view of columnar joints in a basaltic lava flow in Iceland.

Practice with Structures Made by Lava
Query \(\PageIndex{2}\)
Pyroclastic Materials
The pop bottle analogy illustrates another key point about gas bubbles in fluid, which is that the bubbles can propel fluid. In the same way that shaking a pop bottle to make more bubbles will cause pop to gush out when the bottle is opened, gas bubbles can violently propel lava and other materials from a volcano, creating an explosive eruption.
Collectively, loose material thrown from a volcano is referred to as tephra. Individual fragments are referred to in general terms as pyroclasts, so sometimes tephra is also referred to as pyroclastic debris. Pyroclasts are classified according to size.
Volcanic Ash
Particles less than 2 mm in diameter are called volcanic ash. Volcanic ash consists of small mineral grains and glass. Figure 11.16 shows volcanic ash on three scales: in the upper left is ash from the 2010 eruption of Eyjafjallajökull in Iceland. The image was taken with a scanning electron microscope at approximately 1000 times magnification. In the upper right is ash from the 1980 eruption of Mt. St. Helens, collected in Yakima, Washington, about 137 km northeast of Mt. St. Helens. Individual particles are under 1 mm in size. Figure 11.16 (bottom) shows a village near Mt. Merapi in Indonesia dusted in ash after an eruption 2010.

Lapilli
Fragments with dimensions between 2 mm and 64 mm are classified as lapilli. Figure 11.17 (upper left) shows lapilli at the ancient city of Pompeii, which was buried when Mt. Vesuvius erupted in 79 C.E. Figure 11.17 (lower left) is a form of lapilli called Pele’s tears, named after the Hawai’ian diety Pele. Pele’s tears form when droplets of lava cool quickly as they are flung through the air. Rapidly moving through the air may draw the Pele’s tears out into long threads called Pele’s hair (Figure 11.17, right). The dark masses in Figure 11.17 (right) within the Pele’s hair are Pele’s tears.
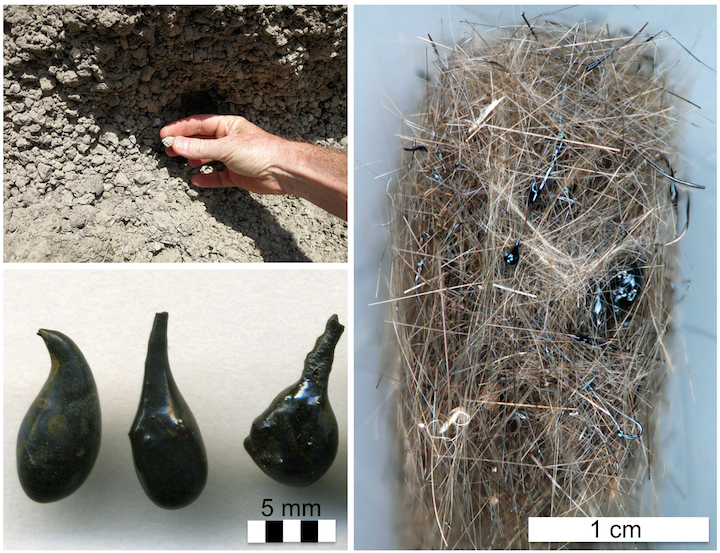
Blocks and Bombs
Fragments larger than 64 mm are classified as blocks or bombs, depending on their origin. Blocks are solid fragments of the volcano that form when an explosive eruption shatters the pre-existing rocks. Figure 11.18 shows one of many blocks from an explosive eruption at the Halema‘uma‘u crater at Kīlauea Volcano in May of 1924. The block has a mass of approximately 7 tonnes and landed 1 km from the crater.

Bombs form when lava is thrown from the volcano and cools as it travels through the air. Traveling through the air may cause the lava to take on a streamlined shape, as with the example in Figure 11.19.

Effects of Gas on Lapilli and Bombs
The presence of gas in erupting lava can cause lapilli and bombs to take on distinctive forms as the lava freezes around the gas bubbles, giving the rocks a vesicular (hole-filled) texture. Pumice (Figure 11.20) forms from gas-filled felsic lava. Figure 11.20 (right), shows a magnified view of the sample on the left. The dark patches in the photograph are mineral crystals that formed in the magma chamber before the lava erupted. Pumice floats on water because some of the holes are completely enclosed, and air-filled.

The mafic counterpart to pumice is scoria (Figure 11.21, left). Mafic lava can also form reticulite (Figure 11.21, right), a rare and fragile rock in which the walls surrounding the bubbles have all burst, leaving behind a delicate network of glass.

Practice with Types of Pyroclastic Material
Query \(\PageIndex{3}\)
References
U. S. Geological Survey (2013) Mt. St. Helens National Volcanic Monument. Retrieved on 11 June 2017. Visit website