3.6: Identifying Minerals
- Page ID
- 6857
\( \newcommand{\vecs}[1]{\overset { \scriptstyle \rightharpoonup} {\mathbf{#1}} } \)
\( \newcommand{\vecd}[1]{\overset{-\!-\!\rightharpoonup}{\vphantom{a}\smash {#1}}} \)
\( \newcommand{\id}{\mathrm{id}}\) \( \newcommand{\Span}{\mathrm{span}}\)
( \newcommand{\kernel}{\mathrm{null}\,}\) \( \newcommand{\range}{\mathrm{range}\,}\)
\( \newcommand{\RealPart}{\mathrm{Re}}\) \( \newcommand{\ImaginaryPart}{\mathrm{Im}}\)
\( \newcommand{\Argument}{\mathrm{Arg}}\) \( \newcommand{\norm}[1]{\| #1 \|}\)
\( \newcommand{\inner}[2]{\langle #1, #2 \rangle}\)
\( \newcommand{\Span}{\mathrm{span}}\)
\( \newcommand{\id}{\mathrm{id}}\)
\( \newcommand{\Span}{\mathrm{span}}\)
\( \newcommand{\kernel}{\mathrm{null}\,}\)
\( \newcommand{\range}{\mathrm{range}\,}\)
\( \newcommand{\RealPart}{\mathrm{Re}}\)
\( \newcommand{\ImaginaryPart}{\mathrm{Im}}\)
\( \newcommand{\Argument}{\mathrm{Arg}}\)
\( \newcommand{\norm}[1]{\| #1 \|}\)
\( \newcommand{\inner}[2]{\langle #1, #2 \rangle}\)
\( \newcommand{\Span}{\mathrm{span}}\) \( \newcommand{\AA}{\unicode[.8,0]{x212B}}\)
\( \newcommand{\vectorA}[1]{\vec{#1}} % arrow\)
\( \newcommand{\vectorAt}[1]{\vec{\text{#1}}} % arrow\)
\( \newcommand{\vectorB}[1]{\overset { \scriptstyle \rightharpoonup} {\mathbf{#1}} } \)
\( \newcommand{\vectorC}[1]{\textbf{#1}} \)
\( \newcommand{\vectorD}[1]{\overrightarrow{#1}} \)
\( \newcommand{\vectorDt}[1]{\overrightarrow{\text{#1}}} \)
\( \newcommand{\vectE}[1]{\overset{-\!-\!\rightharpoonup}{\vphantom{a}\smash{\mathbf {#1}}}} \)
\( \newcommand{\vecs}[1]{\overset { \scriptstyle \rightharpoonup} {\mathbf{#1}} } \)
\( \newcommand{\vecd}[1]{\overset{-\!-\!\rightharpoonup}{\vphantom{a}\smash {#1}}} \)
\(\newcommand{\avec}{\mathbf a}\) \(\newcommand{\bvec}{\mathbf b}\) \(\newcommand{\cvec}{\mathbf c}\) \(\newcommand{\dvec}{\mathbf d}\) \(\newcommand{\dtil}{\widetilde{\mathbf d}}\) \(\newcommand{\evec}{\mathbf e}\) \(\newcommand{\fvec}{\mathbf f}\) \(\newcommand{\nvec}{\mathbf n}\) \(\newcommand{\pvec}{\mathbf p}\) \(\newcommand{\qvec}{\mathbf q}\) \(\newcommand{\svec}{\mathbf s}\) \(\newcommand{\tvec}{\mathbf t}\) \(\newcommand{\uvec}{\mathbf u}\) \(\newcommand{\vvec}{\mathbf v}\) \(\newcommand{\wvec}{\mathbf w}\) \(\newcommand{\xvec}{\mathbf x}\) \(\newcommand{\yvec}{\mathbf y}\) \(\newcommand{\zvec}{\mathbf z}\) \(\newcommand{\rvec}{\mathbf r}\) \(\newcommand{\mvec}{\mathbf m}\) \(\newcommand{\zerovec}{\mathbf 0}\) \(\newcommand{\onevec}{\mathbf 1}\) \(\newcommand{\real}{\mathbb R}\) \(\newcommand{\twovec}[2]{\left[\begin{array}{r}#1 \\ #2 \end{array}\right]}\) \(\newcommand{\ctwovec}[2]{\left[\begin{array}{c}#1 \\ #2 \end{array}\right]}\) \(\newcommand{\threevec}[3]{\left[\begin{array}{r}#1 \\ #2 \\ #3 \end{array}\right]}\) \(\newcommand{\cthreevec}[3]{\left[\begin{array}{c}#1 \\ #2 \\ #3 \end{array}\right]}\) \(\newcommand{\fourvec}[4]{\left[\begin{array}{r}#1 \\ #2 \\ #3 \\ #4 \end{array}\right]}\) \(\newcommand{\cfourvec}[4]{\left[\begin{array}{c}#1 \\ #2 \\ #3 \\ #4 \end{array}\right]}\) \(\newcommand{\fivevec}[5]{\left[\begin{array}{r}#1 \\ #2 \\ #3 \\ #4 \\ #5 \\ \end{array}\right]}\) \(\newcommand{\cfivevec}[5]{\left[\begin{array}{c}#1 \\ #2 \\ #3 \\ #4 \\ #5 \\ \end{array}\right]}\) \(\newcommand{\mattwo}[4]{\left[\begin{array}{rr}#1 \amp #2 \\ #3 \amp #4 \\ \end{array}\right]}\) \(\newcommand{\laspan}[1]{\text{Span}\{#1\}}\) \(\newcommand{\bcal}{\cal B}\) \(\newcommand{\ccal}{\cal C}\) \(\newcommand{\scal}{\cal S}\) \(\newcommand{\wcal}{\cal W}\) \(\newcommand{\ecal}{\cal E}\) \(\newcommand{\coords}[2]{\left\{#1\right\}_{#2}}\) \(\newcommand{\gray}[1]{\color{gray}{#1}}\) \(\newcommand{\lgray}[1]{\color{lightgray}{#1}}\) \(\newcommand{\rank}{\operatorname{rank}}\) \(\newcommand{\row}{\text{Row}}\) \(\newcommand{\col}{\text{Col}}\) \(\renewcommand{\row}{\text{Row}}\) \(\newcommand{\nul}{\text{Nul}}\) \(\newcommand{\var}{\text{Var}}\) \(\newcommand{\corr}{\text{corr}}\) \(\newcommand{\len}[1]{\left|#1\right|}\) \(\newcommand{\bbar}{\overline{\bvec}}\) \(\newcommand{\bhat}{\widehat{\bvec}}\) \(\newcommand{\bperp}{\bvec^\perp}\) \(\newcommand{\xhat}{\widehat{\xvec}}\) \(\newcommand{\vhat}{\widehat{\vvec}}\) \(\newcommand{\uhat}{\widehat{\uvec}}\) \(\newcommand{\what}{\widehat{\wvec}}\) \(\newcommand{\Sighat}{\widehat{\Sigma}}\) \(\newcommand{\lt}{<}\) \(\newcommand{\gt}{>}\) \(\newcommand{\amp}{&}\) \(\definecolor{fillinmathshade}{gray}{0.9}\)Geologists identify minerals by their physical properties. In the field, where geologists may have limited access to advanced technology and powerful machines, they can still identify minerals by testing several physical properties: luster and color, streak, hardness, crystal habit, cleavage and fracture, and some special properties. Only a few common minerals make up the majority of Earth’s rocks and are usually seen as small grains in rocks. Of the several properties used for identifying minerals, it is good to consider which will be most useful for identifying them in small grains surrounded by other minerals.
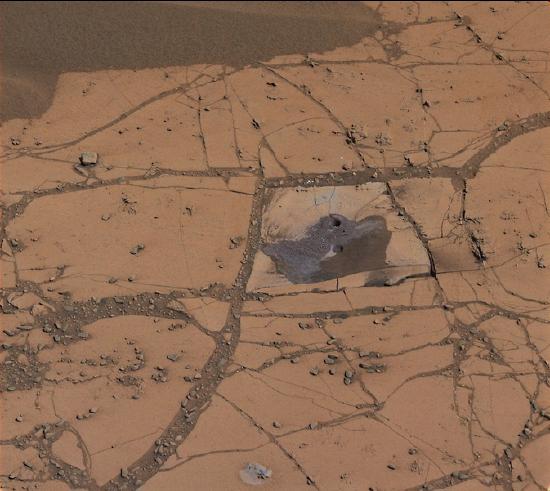
Luster and Color
The first thing to notice about a mineral is its surface appearance, specifically luster and color. Luster describes how the mineral looks. Metallic luster looks like a shiny metal such as chrome, steel, silver, or gold. Submetallic luster has a duller appearance. Pewter, for example, shows submetallic luster.
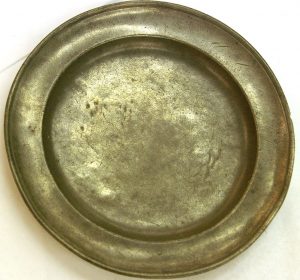
Nonmetallic luster doesn’t look like metal and may be described as vitreous (glassy), earthy, silky, pearly, and other surface qualities. Nonmetallic minerals may be shiny, although their vitreous shine is different from metallic luster. See the table for descriptions and examples of nonmetallic luster.
Luster | Image | Description |
---|---|---|
Vitreous/glassy |
|
Surface is shiny like glass |
Earthy/dull |
![]() |
Dull, like dried mud or clay |
Silky |
|
Soft shine like silk fabric |
Pearly |
|
Like the inside of a clam shell or mother-of-pearl |
Submetallic |
|
Has the appearance of dull metal, like pewter. These minerals would usually still be considered metallic. Submetallic appearance can occur in metallic minerals because of weathering. |
Surface color may be helpful in identifying minerals, although it can be quite variable within the same mineral family. Mineral colors are affected by the main elements as well as impurities in the crystals. These impurities may be rare elements—like manganese, titanium, chromium, or lithium—even other molecules that are not normally part of the mineral formula. For example, the incorporation of water molecules gives quartz, which is normally clear, a milky color.
Some minerals predominantly show a single color. Malachite and azurite are green and blue, respectively, because of their copper content. Other minerals have a predictable range of colors due to elemental substitutions, usually via a solid solution. Feldspars, the most abundant minerals in the earth’s crust, are complex, have solid solution series, and present several colors including pink, white, green, gray and others. Other minerals also come in several colors, influenced by trace amounts of several elements. The same element may show up as different colors, in different minerals. With notable exceptions, color is usually not a definitive property of minerals. For identifying many minerals. a more reliable indicator is a streak, which is the color of the powdered mineral.
Streak
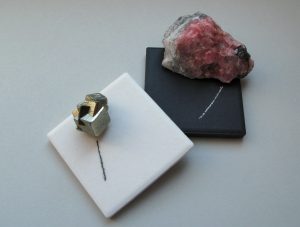
Streak examines the color of a powdered mineral and can be seen when a mineral sample is scratched or scraped on an unglazed porcelain streak plate. A paper page in a field notebook may also be used for the streak of some minerals. Minerals that are harder than the streak plate will not show streak but will scratch the porcelain. For these minerals, a streak test can be obtained by powdering the mineral with a hammer and smearing the powder across a streak plate or notebook paper.
While mineral surface colors and appearances may vary, their streak colors can be diagnostically useful. An example of this property is seen in the iron-oxide mineral hematite. Hematite occurs in a variety of forms, colors, lusters (from shiny metallic silver to earthy red-brown), and different physical appearances. A hematite streak is consistently reddish-brown, no matter what the original specimen looks like. Iron sulfide, or pyrite, is a brassy metallic yellow. Commonly named fool’s gold, pyrite has a characteristic black to greenish-black streak.
Hardness
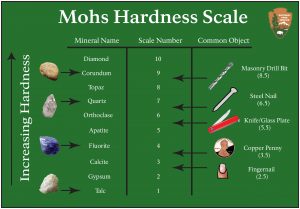
Hardness measures the ability of a mineral to scratch other substances. The Mohs Hardness Scale gives a number showing the relative scratch-resistance of minerals when compared to a standardized set of minerals of increasing hardness. The Mohs scale was developed by German geologist Fredrick Mohs in the early 20th century, although the idea of identifying minerals by hardness goes back thousands of years. Mohs hardness values are determined by the strength of a mineral’s atomic bonds.
The figure shows the minerals associated with specific hardness values, together with some common items readily available for use in field testing and mineral identification. The hardness values run from 1 to 10, with 10 being the hardest; however, the scale is not linear. Diamond defines a hardness of 10 and is actually about four times harder than corundum, which is 9. A steel pocketknife blade, which has a hardness value of 5.5, separates between hard and soft minerals on many mineral identification keys.
Crystal Habit
Minerals can be identified by crystal habit, how their crystals grow and appear in rocks. Crystal shapes are determined by the arrangement of the atoms within the crystal structure. For example, a cubic arrangement of atoms gives rise to a cubic-shaped mineral crystal. Crystal habit refers to typically observed shapes and characteristics; however, they can be affected by other minerals crystallizing in the same rock. When minerals are constrained so they do not develop their typical crystal habit, they are called anhedral. Subhedral crystals are partially formed shapes. For some minerals, characteristic crystal habit is to grow crystal faces even when surrounded by other crystals in the rock. An example is garnet. Minerals grow freely where the crystals are unconstrained and can take characteristic shapes often form crystal faces. A euhedral crystal has a perfectly formed, unconstrained shape. Some minerals crystallize in such tiny crystals, they do not show a specific crystal habit to the naked eye. Other minerals, like pyrite, can have an array of different crystal habits, including cubic, dodecahedral, octahedral, and massive. The table lists typical crystal habits of various minerals.
Habit | Image | Examples |
---|---|---|
Bladed
long and flat crystals |
|
kyanite, amphibole, gypsum |
Botryoidal/mammillary
blobby, circular crystals |
|
hematite, malachite, smithsonite |
Coating/laminae/druse
crystals that are small and coat surfaces |
|
quartz, calcite, malachite, azurite |
Cubic
cube-shaped crystals |
![]() |
pyrite, galena, halite |
Dodecahedral
12-sided polygon shapes |
|
garnet, pyrite |
Dendritic
branching crystals |
|
Mn-oxides, copper, gold |
Equant
crystals that do not have a long direction |
|
olivine, garnet, pyroxene |
Fibrous
thin, very long crystals |
|
serpentine, amphibole, zeolite |
Layered, sheets
stacked, very thin, flat crystals |
![]() |
mica (biotite, muscovite, etc.) |
Lenticular/platy
crystals that are plate-like |
|
selenite roses, wulfenite, calcite |
Hexagonal
crystals with six sides |
![]() |
quartz, hanksite, corundum |
Massive/granular
Crystals with no obvious shape, microscopic crystals |
![]() |
limonite, pyrite, azurite, bornite |
Octahedral
4-sided double pyramid crystals |
|
diamond, fluorite, magnetite, pyrite |
Prismatic/columnar
very long, cylindrical crystals |
|
tourmaline, beryl, barite |
Radiating
crystals that grow from a point and fan-out |
|
pyrite “suns”, pyrophyllite |
Rhombohedral
crystals shaped like slanted cubes |
![]() |
calcite, dolomite |
Tabular/blocky/stubby
sharp-sided crystals with no long direction |
|
feldspar, pyroxene, calcite |
Tetrahedral
three-sided, pyramid-shaped crystals |
|
magnetite, spinel, tetrahedrite |
Another crystal habit that may be used to identify minerals is striations, which are dark and light parallel lines on a crystal face. Twinning is another, which occurs when the crystal structure replicates in mirror images along certain directions in the crystal.
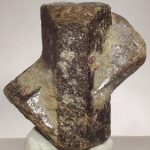
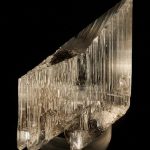
Striations and twinning are related properties in some minerals including plagioclase feldspar. Striations are optical lines on a cleavage surface. Because of twinning in the crystal, striations show up on one of the two cleavage faces of the plagioclase crystal.
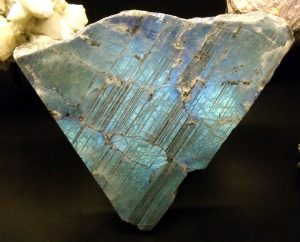
Cleavage and Fracture
Minerals often show characteristic patterns of breaking along specific cleavage planes or show characteristic fracture patterns. Cleavage planes are smooth, flat, parallel planes within the crystal. The cleavage planes may show as reflective surfaces on the crystal, as parallel cracks that penetrate into the crystal, or show on the edge or side of the crystal as a series of steps like rice terraces. Cleavage arises in crystals where the atomic bonds between atomic layers are weaker along some directions than others, meaning they will break preferentially along these planes. Because they develop on atomic surfaces in the crystal, cleavage planes are optically smooth and reflect light, although the actual break on the crystal may appear jagged or uneven. In such cleavages, the cleavage surface may appear like rice terraces on a mountainside that all reflect sunlight from a particular sun angle. Some minerals have a strong cleavage, some minerals only have weak cleavage or do not typically demonstrate cleavage.
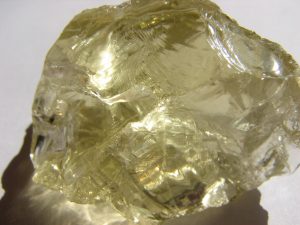
For example, quartz and olivine rarely show cleavage and typically break into conchoidal fracture patterns.
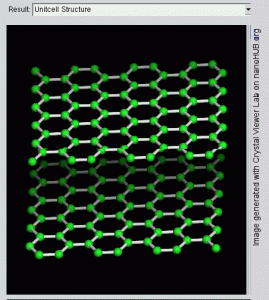
Graphite has its carbon atoms arranged into layers with relatively strong bonds within the layer and very weak bonds between the layers. Thus graphite cleaves readily between the layers and the layers slide easily over one another giving graphite its lubricating quality.
Mineral fracture surfaces may be rough, uneven, or show a conchoidal fracture. Uneven fracture patterns are described as irregular, splintery, fibrous. A conchoidal fracture has a smooth, curved surface like a shallow bowl or conch shell, often with curved ridges. Natural volcanic glass, called obsidian, breaks with this characteristic conchoidal pattern
To work with cleavage, it is important to remember that cleavage is a result of bonds separating along planes of atoms in the crystal structure. On some minerals, cleavage planes may be confused with crystal faces. This will usually not be an issue for crystals of minerals that grew together within rocks. The act of breaking the rock to expose a fresh face will most likely break the crystals along cleavage planes. Some cleavage planes are parallel with crystal faces but many are not. Cleavage planes are smooth, flat, parallel planes within the crystal. The cleavage planes may show as parallel cracks that penetrate into the crystal (see amphibole below), or show on the edge or side of the crystal as a series of steps like rice terraces. For some minerals, characteristic crystal habit is to grow crystal faces even when surrounded by other crystals in rock. An example is garnet. Minerals grow freely where the crystals are unconstrained and can take characteristic shapes often form crystal faces (see quartz below).
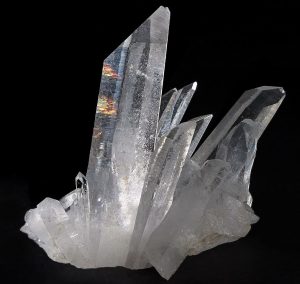
In some minerals, distinguishing cleavage planes from crystal faces may be challenging for the student. Understanding the nature of cleavage and referring to the number of cleavage planes and cleavage angles on identification keys should provide the student with enough information to distinguish cleavages from crystal faces. Cleavage planes may show as multiple parallel cracks or flat surfaces on the crystal. Cleavage planes may be expressed as a series of steps like terraced rice paddies. See the cleavage surfaces on galena above or plagioclase below. Cleavage planes arise from the tendency of mineral crystals to break along specific planes of weakness within the crystal favored by atomic arrangements. The number of cleavage planes, the quality of the cleavage surfaces, and the angles between them are diagnostic for many minerals and cleavage is one of the most useful properties for identifying minerals. Learning to recognize cleavage is an especially important and useful skill in studying minerals.
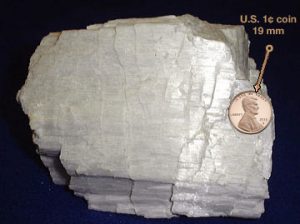
As an identification property of minerals, cleavage is usually given in terms of the quality of the cleavage (perfect, imperfect, or none), the number of cleavage surfaces, and the angles between the surfaces. The most common number of cleavage plane directions in the common rock-forming minerals are one perfect cleavage (as in mica), two cleavage planes (as in feldspar, pyroxene, and amphibole), and three cleavage planes (as in halite, calcite, and galena). One perfect cleavage (as in mica) develops on the top and bottom of the mineral specimen with many parallel cracks showing on the sides but no angle of intersection. Two cleavage planes intersect at an angle. Common cleavage angles are 60°, 75°, 90°, and 120°. Amphibole has two cleavage planes at 60° and 120°. Galena and halite have three cleavage planes at 90° (cubic cleavage). Calcite cleaves readily in three directions producing a cleavage figure called a rhomb that looks like a cube squashed over toward one corner giving rise to the approximately 75° cleavage angles. Pyroxene has an imperfect cleavage with two planes at 90°.
Cleavages on Common Rock-Forming Minerals
- Quartz—none (conchoidal fracture)
- Olivine—none (conchoidal fracture)
- Mica—1 perfect
- Feldspar—2 perfect at 90°
- Pyroxene—2 imperfect at 90°
- Amphibole—2 perfect at 60°/120°
- Calcite—3 perfect at approximately 75°
- Halite, galena, pyrite—3 perfect at 90°
Special Properties
Special properties are unique and identifiable characteristics used to identify minerals or that allow some minerals to be used for special purposes. Ulexite has a fiber-optic property that can project images through the crystal-like a high-definition television screen (see figure). A simple identifying special property is taste, such as the salty flavor of halite or common table salt (NaCl). Sylvite is potassium chloride (KCl) and has a more bitter taste.
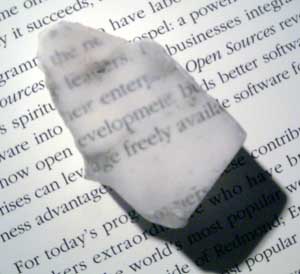
Another property geologists may use to identify minerals is a property related to density called specific gravity. Specific gravity measures the weight of a mineral specimen relative to the weight of an equal volume of water. The value is expressed as a ratio between the mineral and water weights. To measure specific gravity, a mineral specimen is first weighed in grams then submerged in a graduated cylinder filled with pure water at room temperature. The rise in water level is noted using the cylinder’s graduated scale. Since the weight of water at room temperature is 1 gram per cubic centimeter, the ratio of the two-weight numbers gives the specific gravity. Specific gravity is easy to measure in the laboratory but is less useful for mineral identification in the field than other more easily observed properties, except in a few rare cases such as the very dense galena or native gold. The high density of these minerals gives rise to a qualitative property called “heft.” Experienced geologists can roughly assess specific gravity by heft, a subjective quality of how heavy the specimen feels in one’s hand relative to its size.
A simple test for identifying calcite and dolomite is to drop a bit of dilute hydrochloric acid (10-15% HCl) on the specimen. If the acid drop effervesces or fizzes on the surface of the rock, the specimen is calcite. If it does not, the specimen is scratched to produce a small amount of powder and test with acid again. If the acid drop fizzes slowly on the powdered mineral, the specimen is dolomite. The difference between these two minerals can be seen in the video. Geologists who work with carbonate rocks carry a small dropper bottle of dilute HCl in their field kit. Vinegar, which contains acetic acid, can be used for this test and is used to distinguish non-calcite fossils from limestone. While acidic, vinegar produces less of a fizzing reaction because acetic acid is a weaker acid.
Some iron-oxide minerals are magnetic and are attracted to magnets. A common name for naturally magnetic iron oxide is lodestone. Others include magnetite (Fe3O4) and ilmenite (FeTiO3). Magnetite is strongly attracted to magnets and can be magnetized. Ilmenite and some types of hematite are weakly magnetic.
Some minerals and mineraloids scatter light via a phenomenon called iridescence. This property occurs in labradorite (a variety of plagioclase) and opal. It is also seen in biologically created substances like pearls and seashells. Cut diamonds show iridescence and the jeweler’s diamond cut is designed to maximize this property.
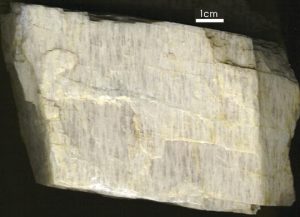
Striations on mineral cleavage faces are an optical property that can be used to separate plagioclase feldspar from potassium feldspar (K-spar). A process called twinning creates parallel zones in the crystal that are repeating mirror images. The actual cleavage angle in plagioclase is slightly different than 90o and the alternating mirror images in these twinned zones produce a series of parallel lines on one of plagioclase’s two cleavage faces. Light reflects off these twinned lines at slightly different angles which then appear as light and dark lines called striations on the cleavage surface. Potassium feldspar does not exhibit twinning or striations but may show linear features called exsolution lamellae, also known as perthitic lineation or simply perthite. Because sodium and potassium do not fit into the same feldspar crystal structure, the lines are created by small amounts of sodium feldspar (albite) separating from the dominant potassium feldspar (K-spar) within the crystal structure. The two different feldspars crystallize out into roughly parallel zones within the crystal, which are seen as these linear markings.
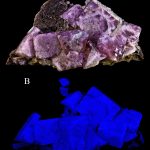
One of the most interesting special mineral properties is fluorescence. Certain minerals, or trace elements within them, give off visible light when exposed to ultraviolet radiation or black light. Many mineral exhibits have a fluorescence room equipped with black lights so this property can be observed. An even rarer optical property is phosphorescence. Phosphorescent minerals absorb light and then slowly release it, much like a glow-in-the-dark sticker.