4.4: Qualitative Aspects of Groundwater and Groundwater Flow
- Page ID
- 13471
\( \newcommand{\vecs}[1]{\overset { \scriptstyle \rightharpoonup} {\mathbf{#1}} } \)
\( \newcommand{\vecd}[1]{\overset{-\!-\!\rightharpoonup}{\vphantom{a}\smash {#1}}} \)
\( \newcommand{\id}{\mathrm{id}}\) \( \newcommand{\Span}{\mathrm{span}}\)
( \newcommand{\kernel}{\mathrm{null}\,}\) \( \newcommand{\range}{\mathrm{range}\,}\)
\( \newcommand{\RealPart}{\mathrm{Re}}\) \( \newcommand{\ImaginaryPart}{\mathrm{Im}}\)
\( \newcommand{\Argument}{\mathrm{Arg}}\) \( \newcommand{\norm}[1]{\| #1 \|}\)
\( \newcommand{\inner}[2]{\langle #1, #2 \rangle}\)
\( \newcommand{\Span}{\mathrm{span}}\)
\( \newcommand{\id}{\mathrm{id}}\)
\( \newcommand{\Span}{\mathrm{span}}\)
\( \newcommand{\kernel}{\mathrm{null}\,}\)
\( \newcommand{\range}{\mathrm{range}\,}\)
\( \newcommand{\RealPart}{\mathrm{Re}}\)
\( \newcommand{\ImaginaryPart}{\mathrm{Im}}\)
\( \newcommand{\Argument}{\mathrm{Arg}}\)
\( \newcommand{\norm}[1]{\| #1 \|}\)
\( \newcommand{\inner}[2]{\langle #1, #2 \rangle}\)
\( \newcommand{\Span}{\mathrm{span}}\) \( \newcommand{\AA}{\unicode[.8,0]{x212B}}\)
\( \newcommand{\vectorA}[1]{\vec{#1}} % arrow\)
\( \newcommand{\vectorAt}[1]{\vec{\text{#1}}} % arrow\)
\( \newcommand{\vectorB}[1]{\overset { \scriptstyle \rightharpoonup} {\mathbf{#1}} } \)
\( \newcommand{\vectorC}[1]{\textbf{#1}} \)
\( \newcommand{\vectorD}[1]{\overrightarrow{#1}} \)
\( \newcommand{\vectorDt}[1]{\overrightarrow{\text{#1}}} \)
\( \newcommand{\vectE}[1]{\overset{-\!-\!\rightharpoonup}{\vphantom{a}\smash{\mathbf {#1}}}} \)
\( \newcommand{\vecs}[1]{\overset { \scriptstyle \rightharpoonup} {\mathbf{#1}} } \)
\( \newcommand{\vecd}[1]{\overset{-\!-\!\rightharpoonup}{\vphantom{a}\smash {#1}}} \)
\(\newcommand{\avec}{\mathbf a}\) \(\newcommand{\bvec}{\mathbf b}\) \(\newcommand{\cvec}{\mathbf c}\) \(\newcommand{\dvec}{\mathbf d}\) \(\newcommand{\dtil}{\widetilde{\mathbf d}}\) \(\newcommand{\evec}{\mathbf e}\) \(\newcommand{\fvec}{\mathbf f}\) \(\newcommand{\nvec}{\mathbf n}\) \(\newcommand{\pvec}{\mathbf p}\) \(\newcommand{\qvec}{\mathbf q}\) \(\newcommand{\svec}{\mathbf s}\) \(\newcommand{\tvec}{\mathbf t}\) \(\newcommand{\uvec}{\mathbf u}\) \(\newcommand{\vvec}{\mathbf v}\) \(\newcommand{\wvec}{\mathbf w}\) \(\newcommand{\xvec}{\mathbf x}\) \(\newcommand{\yvec}{\mathbf y}\) \(\newcommand{\zvec}{\mathbf z}\) \(\newcommand{\rvec}{\mathbf r}\) \(\newcommand{\mvec}{\mathbf m}\) \(\newcommand{\zerovec}{\mathbf 0}\) \(\newcommand{\onevec}{\mathbf 1}\) \(\newcommand{\real}{\mathbb R}\) \(\newcommand{\twovec}[2]{\left[\begin{array}{r}#1 \\ #2 \end{array}\right]}\) \(\newcommand{\ctwovec}[2]{\left[\begin{array}{c}#1 \\ #2 \end{array}\right]}\) \(\newcommand{\threevec}[3]{\left[\begin{array}{r}#1 \\ #2 \\ #3 \end{array}\right]}\) \(\newcommand{\cthreevec}[3]{\left[\begin{array}{c}#1 \\ #2 \\ #3 \end{array}\right]}\) \(\newcommand{\fourvec}[4]{\left[\begin{array}{r}#1 \\ #2 \\ #3 \\ #4 \end{array}\right]}\) \(\newcommand{\cfourvec}[4]{\left[\begin{array}{c}#1 \\ #2 \\ #3 \\ #4 \end{array}\right]}\) \(\newcommand{\fivevec}[5]{\left[\begin{array}{r}#1 \\ #2 \\ #3 \\ #4 \\ #5 \\ \end{array}\right]}\) \(\newcommand{\cfivevec}[5]{\left[\begin{array}{c}#1 \\ #2 \\ #3 \\ #4 \\ #5 \\ \end{array}\right]}\) \(\newcommand{\mattwo}[4]{\left[\begin{array}{rr}#1 \amp #2 \\ #3 \amp #4 \\ \end{array}\right]}\) \(\newcommand{\laspan}[1]{\text{Span}\{#1\}}\) \(\newcommand{\bcal}{\cal B}\) \(\newcommand{\ccal}{\cal C}\) \(\newcommand{\scal}{\cal S}\) \(\newcommand{\wcal}{\cal W}\) \(\newcommand{\ecal}{\cal E}\) \(\newcommand{\coords}[2]{\left\{#1\right\}_{#2}}\) \(\newcommand{\gray}[1]{\color{gray}{#1}}\) \(\newcommand{\lgray}[1]{\color{lightgray}{#1}}\) \(\newcommand{\rank}{\operatorname{rank}}\) \(\newcommand{\row}{\text{Row}}\) \(\newcommand{\col}{\text{Col}}\) \(\renewcommand{\row}{\text{Row}}\) \(\newcommand{\nul}{\text{Nul}}\) \(\newcommand{\var}{\text{Var}}\) \(\newcommand{\corr}{\text{corr}}\) \(\newcommand{\len}[1]{\left|#1\right|}\) \(\newcommand{\bbar}{\overline{\bvec}}\) \(\newcommand{\bhat}{\widehat{\bvec}}\) \(\newcommand{\bperp}{\bvec^\perp}\) \(\newcommand{\xhat}{\widehat{\xvec}}\) \(\newcommand{\vhat}{\widehat{\vvec}}\) \(\newcommand{\uhat}{\widehat{\uvec}}\) \(\newcommand{\what}{\widehat{\wvec}}\) \(\newcommand{\Sighat}{\widehat{\Sigma}}\) \(\newcommand{\lt}{<}\) \(\newcommand{\gt}{>}\) \(\newcommand{\amp}{&}\) \(\definecolor{fillinmathshade}{gray}{0.9}\)Figure 4-14 shows a vertical cross section through a representative area of the Earth’s surface, showing some of the important features of distribution of groundwater. The part of the subsurface lying above the groundwater table is called the vadose zone or aerated zone or unsaturated zone. Most of the time, the pore spaces in the vadose zone are occupied mostly by air (plus locally generated gases); only right after a heavy rain are the pore spaces filled with downward- percolating water. The part of the subsurface zone lying below the groundwater table is called the phreatic zone or the saturated zone. In the phreatic zone the pore spaces are always filled with water.
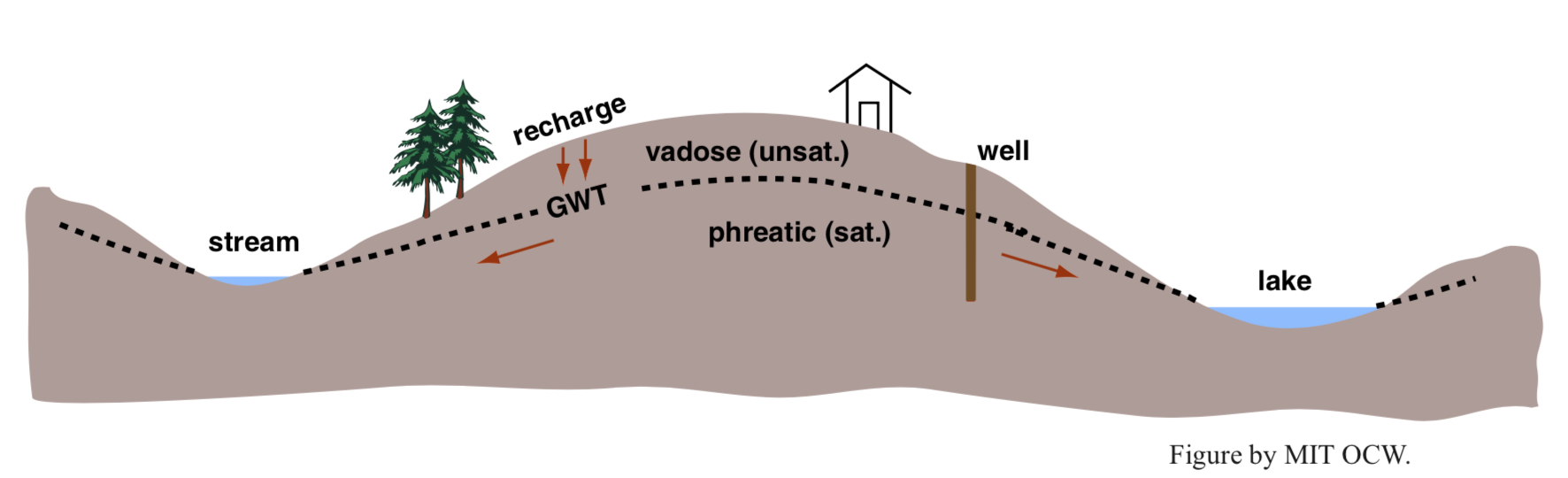
In reality the interface between the vadose zone and the phreatic zone (that is, the groundwater table) is not a sharp and well-defined surface: it’s a fuzzy zone of transition. That’s because of capillary rise of water up into the pore spaces of the lowermost part of the phreatic zone. (See the background section on surface tension and capillarity earlier in the chapter.) The zone of partial saturation is called the capillary fringe. Its thickness ranges from just a centimeter or two, in coarse gravels, to as much as a couple of meters, in fine silt-rich sediments. The reason that there is a gradation in the degree of saturation is that, owing to the spread of particle sizes, adjacent pore spaces vary widely in their effective size.
The topography of the groundwater table mimics the topography of the land surface itself: it’s high under hills and low under valleys. But the topography of the groundwater table is more subdued than that of the land surface, because the depth to the groundwater table is greatest under hills and least under valleys. In fact, the groundwater table intersects the land surface at rivers and lakes and springs. This should not be surprising to you if you think of rivers and lakes as just the places where the permanently saturated zone emerges from beneath the mantle of porous medium!
There’s no necessary relationship between the groundwater table (the surface of contact between the vadose zone and the phreatic zone), on the one hand, and the contact between bedrock and regolith, on the other hand. In areas with a relatively thin mantle of regolith and a relatively deep water table, the water table lies mostly within bedrock (Figure 4-15A). In areas with a relatively thick mantle of regolith and a relatively shallow water table, however, the water table lies mostly within the regolith (Figure 4-15B). In New England, both extremes are common, basically because of the highly variable depth of regolith resulting from glacial erosion, transportation, and deposition of regolith during the last Ice Age.
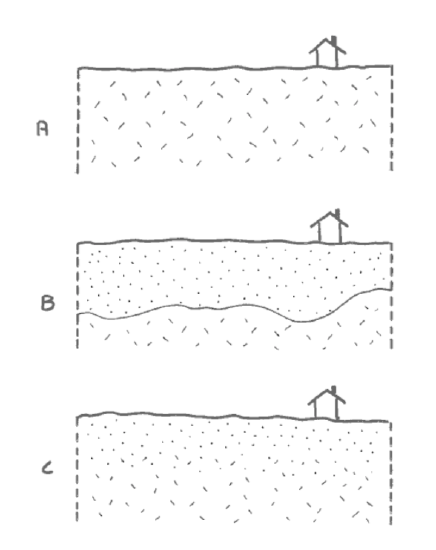
Figure 4-16 shows a record of the level of the water table in a well located as shown in Figure 4-14 during a representative year. In this area, the water table fluctuates vertically by about ten feet in the course of the year. Fluctuations might be considerably smaller or larger than that, depending partly on the variability of rainfall but also very importantly on the permeability of the subsurface material. Obviously, the water table tends to be high during spring and fall rainy periods and low during summer droughts. But the water table tends to be low during the winter also, because the ground at the surface freezes to a depth of a meter or even more, preventing, or at least impeding, recharge, while the groundwater at greater depths continues to flow and thus lower the water table. (The term recharge is used to describe replenishment of groundwater in a subsurface region from which groundwater was previously withdrawn, either naturally or by human activities.) Another noteworthy thing about the record in Figure 4-16 is that the water table rises faster than it falls. This is because recharge involves percolation vertically downward through a relatively thin layer of porous material, whereas drainage involves slower groundwater movement through long distances down low gradients of hydraulic head.
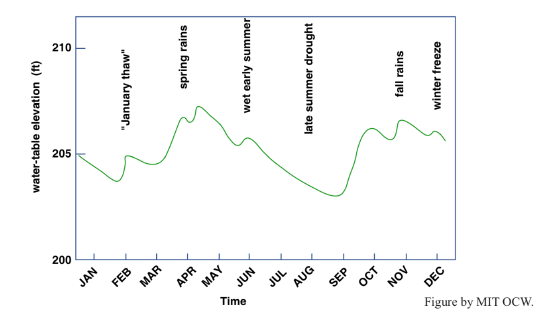
Here’s an incidental note on the depth of water wells. Usually a well is drilled far below the level of the local water table, for two reasons:
- to ensure that the bottom of the well stays below the water table even during severe droughts;
- to get below the level of polluted near-surface waters (but the problem is that even the deeper waters don’t stay unpolluted forever!).
The prices one pays for deeper wells (aside from the dollar cost per foot of well) are:
- generally, the deeper the well the smaller the porosity and permeability of the medium, and so the lower the rate of flow into the well;
- The slower the flow into the well, the longer the residence time of the groundwater in the porous medium, so the longer the time available for uptake of ions from the medium, so the harder the water.
An aquifer is any body of porous material in the Earth (rock or regolith) with sufficient volume and connected porosity to yield appreciable water to wells or springs. Aquifers contain relatively large drainable porosity, relatively large volume, and relatively high permeability. The concept of an aquifer is a loose one, partly because of those sneaky words appreciable and relatively: The term is used for any volume of subsurface material that’s a good producer, or a potentially good producer, of water, relative to surrounding volumes of subsurface material. The minimum lateral dimensions of what are called aquifers might be as little as tens of meters, and the minimum vertical dimensions might be as little as some meters. Large aquifers, however, might have lateral dimensions of hundreds of kilometers and vertical dimensions of many tens of meters.
The opposite concept is that of an aquiclude: any body of subsurface material through which water can move at only negligible rates, or at least at rates much smaller than through adjacent aquifers. Also, the term aquitard is used for any body of subsurface material through which groundwater travels slowly, relative to some adjacent aquifer, but not so slowly as to be negligible, as in an aquiclude.
Aquifers can be classified into four kinds (Figure 4-17):
- unconfined aquifer: an aquifer in which the groundwater is in direct contact with the overlying atmosphere through connected pore space.
- confined aquifer: an aquifer overlain by an aquiclude so that it is not in contact with the atmosphere except in some upstream area of recharge.
- leaky aquifer: a confined aquifer whose overlying aquiclude allows some non-negligible passage of groundwater into or out of the aquifer.
- perched aquifer: an unconfined aquifer present above a shallow and laterally restricted aquiclude.
Aquifers and aquicludes can exist on regional as well as local scales. Figure 4-18 shows a cross section through such a system. A highly porous and permeable sedimentary formation, like a well sorted and poorly cemented sandstone, is overlain by a highly impermeable shale in a large region with very low angles of dip of the formations. Wells may tap the confined aquifer at distances of hundreds of kilometers from the recharge area, where the aquifer formation is exposed at the surface. Note that there’s also a much shallower unconfined aquifer that derives its water much more locally.
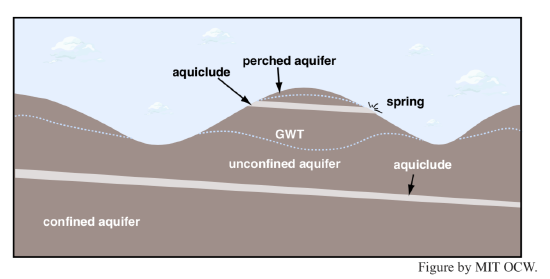
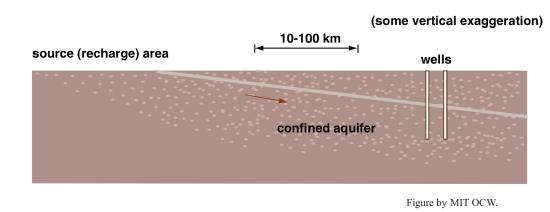
If the surface slope of the region is greater than the slope of the surface of hydraulic head associated with the regional confined aquifer, a well drilled to the confined aquifer will produce a water flow at the ground surface, with no need for pumping (Figure 4-19). Such a well is called an artesian well. Figure 2-20 shows a homey analogy in which you water your garden with a hose leading from an elevated tank of water. In this situation, the nozzle of the hose is a kind of artesian well. And, in a broad sense, if you live in an area with a central municipal water supply all of the faucets in your home or apartment are artesian wells!
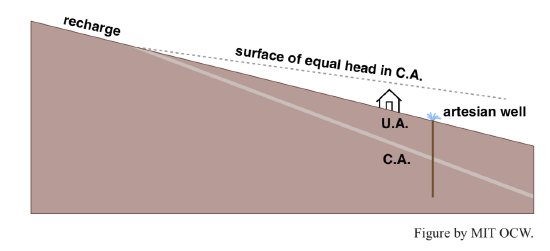
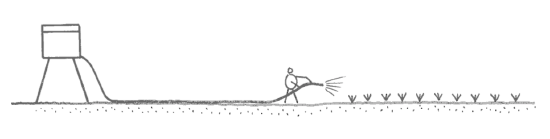
Here’s one more concept that’s useful in dealing with groundwater supplies: The specific yield of an aquifer is the ratio of the volume of water that drains out of the aquifer (when the groundwater table is lowered) to the total volume of the aquifer subjected to drainage. The specific yield obviously depends on the porosity of the aquifer, but it also depends on the size of the pore spaces, because some water always adheres to the surfaces of the solid materials of the aquifer, both as thin films on surfaces and as fillets in reentrants, and the smaller the size of the pore spaces, the greater the percentage of the total porosity that remains occupied by this adhering water. This concept of specific yield is analogous to that of the field capacity of a soil.
I haven’t said anything yet about the patterns of flow within a typical aquifer. The complexities lie in flow through deep unconfined aquifers, because shallow unconfined aquifers (as in Figure 4-12) or confined aquifers (as in Figure 4-18) can be treated approximately as flow in rivers or closed ducts. The patterns of flow in a porous medium are a response to the spatial distribution of gradients of dynamic pressure. No simple statement about the patterns of motion can be made without setting up the geometry of the problem and solving the equation (essentially Newton’s second law written in the appropriate form) that governs the motion.
Figure 4-21 shows qualitatively the flow patterns in a typical deep unconfined aquifer in an area of irregular surface topography. Just below the water table in areas where the water table is sloping, the flow is as you might expect: downward and parallel to the water table. But near the crest of the water table the flow is nearly vertically downward, and directly beneath where the water table merges with rivers and lakes the flow is nearly vertically upward. At points deep within the aquifer, the directions of flow bear no obvious resemblance to the surface topography of the water table.
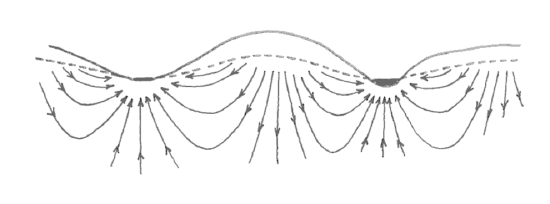