5.5: Volcanism
- Last updated
- Save as PDF
- Page ID
- 15780
- Chris Johnson, Matthew D. Affolter, Paul Inkenbrandt, & Cam Mosher
- Salt Lake Community College via OpenGeology
When magma emerges onto the Earth’s surface, the molten rock is called lava. A volcano is a type of land formation created when lava solidifies into rock. Volcanoes have been an important part of human society for centuries, though their understanding has greatly increased as our understanding of plate tectonics has made them less mysterious. This section describes volcano location, type, hazards, and monitoring.
Distribution and Tectonics
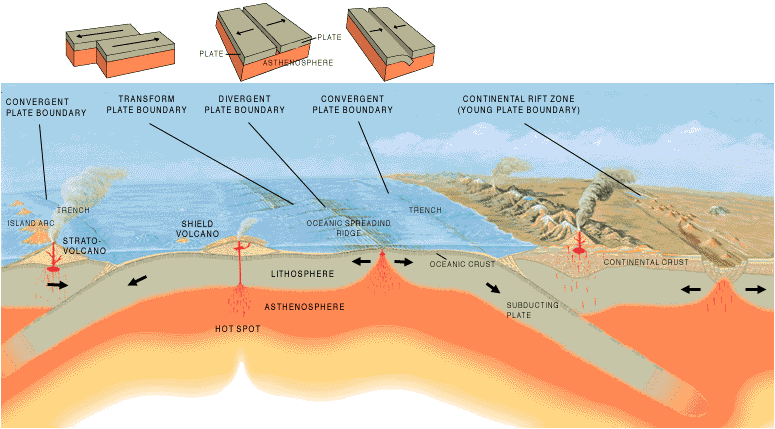
Most volcanoes are interplate volcanoes. Interplate volcanoes are located at active plate boundaries created by volcanism at mid-ocean ridges, subduction zones, and continental rifts. The prefix “inter-“ means between. Some volcanoes are intraplate volcanoes. The prefix “intra-“ means within, and intraplate volcanoes are located within tectonic plates, far removed from plate boundaries. Many intraplate volcanoes are formed by hotspots.
Volcanoes at Mid-Ocean Ridges
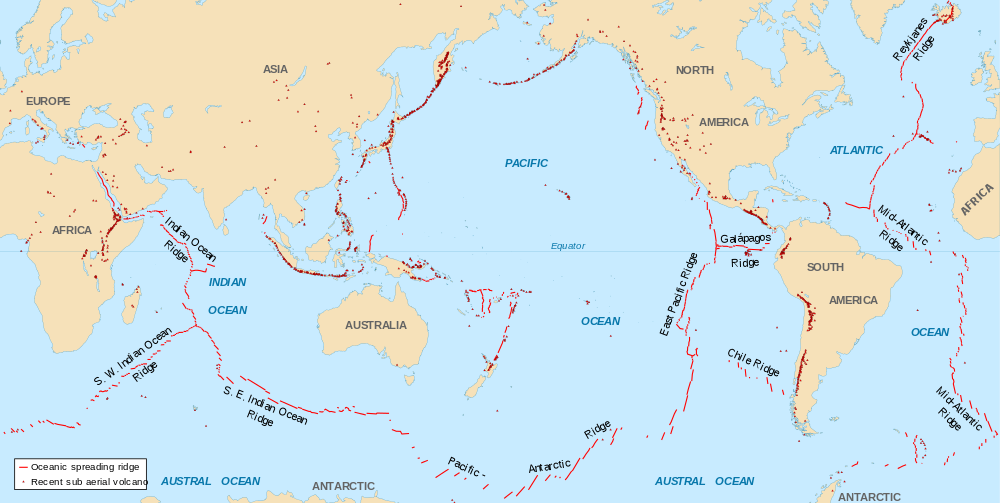
Most volcanism on Earth occurs on the ocean floor along mid-ocean ridges, a type of divergent plate boundary (see Chapter 2). These interplate volcanoes are also the least observed and famous since most of them are located under 3,000-4,500 m (10,000-15,000 ft) of the ocean and the eruptions are slow, gentle, and oozing. One exception is the interplate volcanoes of Iceland. The diverging and thinning oceanic plates allow hot mantle rock to rise, releasing pressure and causing decompression melting. Ultramafic mantle rock, consisting largely of peridotite, partially melts and generates magma that is basaltic. Because of this, almost all volcanoes on the ocean floor are basaltic.
In fact, most oceanic lithosphere is basaltic near the surface, with phaneritic gabbro and ultramafic peridotite underneath [10].
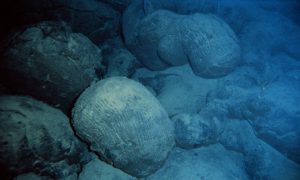
When basaltic lava erupts underwater it emerges in small explosions and/or forms pillow-shaped structures called pillow basalts. These seafloor eruptions enable entire underwater ecosystems to thrive in the deep ocean around mid-ocean ridges. This ecosystem exists around tall vents emitting black, hot mineral-rich water called deep-sea hydrothermal vents, also known as black smokers.
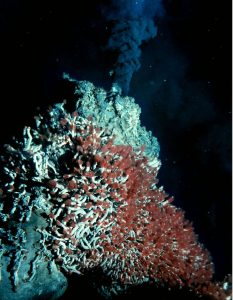
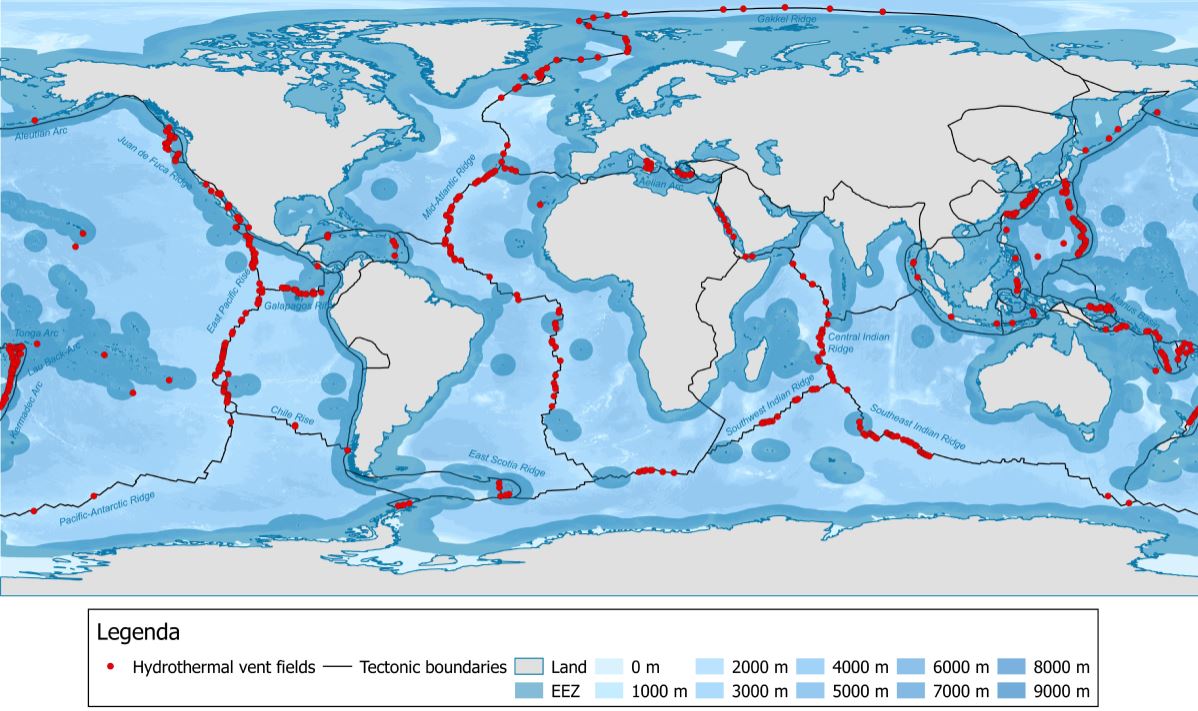
Without sunlight to support photosynthesis, these organisms instead utilize a process called chemosynthesis. Certain bacteria are able to turn hydrogen sulfide (H2S), a gas that smells like rotten eggs, into life-supporting nutrients and water. Larger organisms may eat these bacteria or absorb nutrients and water produced by bacteria living symbiotically inside their bodies [11]. The three videos show some of the ecosystems found around deep-sea hydrothermal vents.
Volcanoes at Subduction Zones
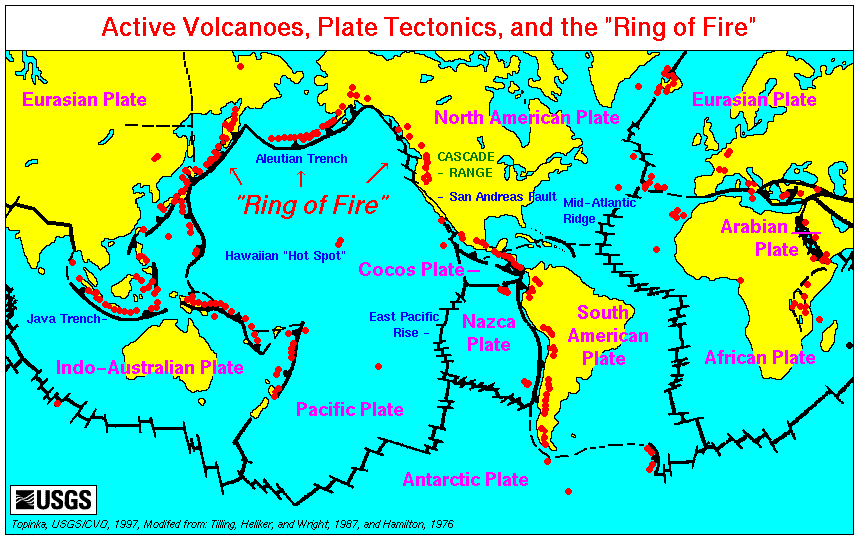
The second most commonly found location for volcanism is adjacent to subduction zones, a type of convergent plate boundary (see Chapter 2). The process of subduction expels water from hydrated minerals in the descending slab, which causes flux melting in the overlying mantle rock. Because subduction volcanism occurs in a volcanic arc, the thickened crust promotes partial melting and magma differentiation. These evolve the mafic magma from the mantle into more silica-rich magma. The Ring of Fire surrounding the Pacific Ocean, for example, is dominated by subduction-generated eruptions of mostly silica-rich lava; the volcanoes and plutons consist largely of intermediate-to-felsic rock such as andesite, rhyolite, pumice, and tuff.
Volcanoes at Continental Rifts
Some volcanoes are created at continental rifts, where crustal thinning is caused by diverging lithospheric plates, such as the East African Rift Basin in Africa. Volcanism caused by crustal thinning without continental rifting is found in the Basin and Range Province in North America. In this location, volcanic activity is produced by rising magma that stretches the overlying crust (see figure). Lower crust or upper mantle material rises through the thinned crust, releases pressure, and undergoes decompression-induced partial melting. This magma is less dense than the surrounding rock and continues to rise through the crust to the surface, erupting as basaltic lava. These eruptions usually result in flood basalts, cinder cones, and basaltic lava flows (see video). Relatively young cinder cones of basaltic lava can be found in south-central Utah, in the Black Rock Desert Volcanic Field, which is part of the zone of Basin and Range crustal extension. These Utah cinder cones and lava flows started erupting around 6 million years ago, with the last eruption occurring 720 years ago [12].
Hotspots
Hotspots are the main source of intraplate volcanism. Hotspots occur when lithospheric plates glide over a hot mantle plume, an ascending column of solid heated rock originating from deep within the mantle. The mantle plume generates melts as material rises, with the magma rising even more. When the ascending magma reaches the lithospheric crust, it spreads out into a mushroom-shaped head that is tens to hundreds of kilometers across.
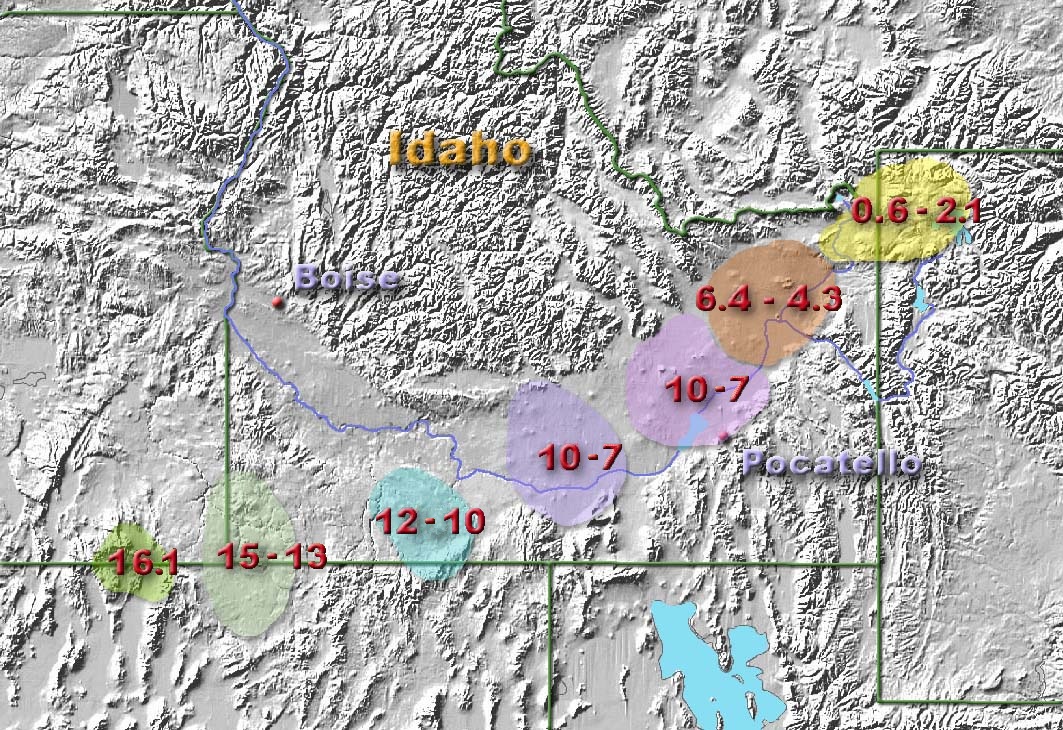
Since most mantle plumes are located beneath the oceanic lithosphere, the early stages of intraplate volcanism typically take place underwater. Over time, basaltic volcanoes may build up from the seafloor into islands, such as the Hawaiian Islands [13]. Where a hotspot is found under a continental plate, contact with the hot mafic magma may cause the overlying felsic rock to melt and mix with the mafic material below, forming intermediate magma. Or the felsic magma may continue to rise, and cool into a granitic batholith or erupt as a felsic volcano. The Yellowstone caldera is an example of hotspot volcanism that resulted in an explosive eruption.
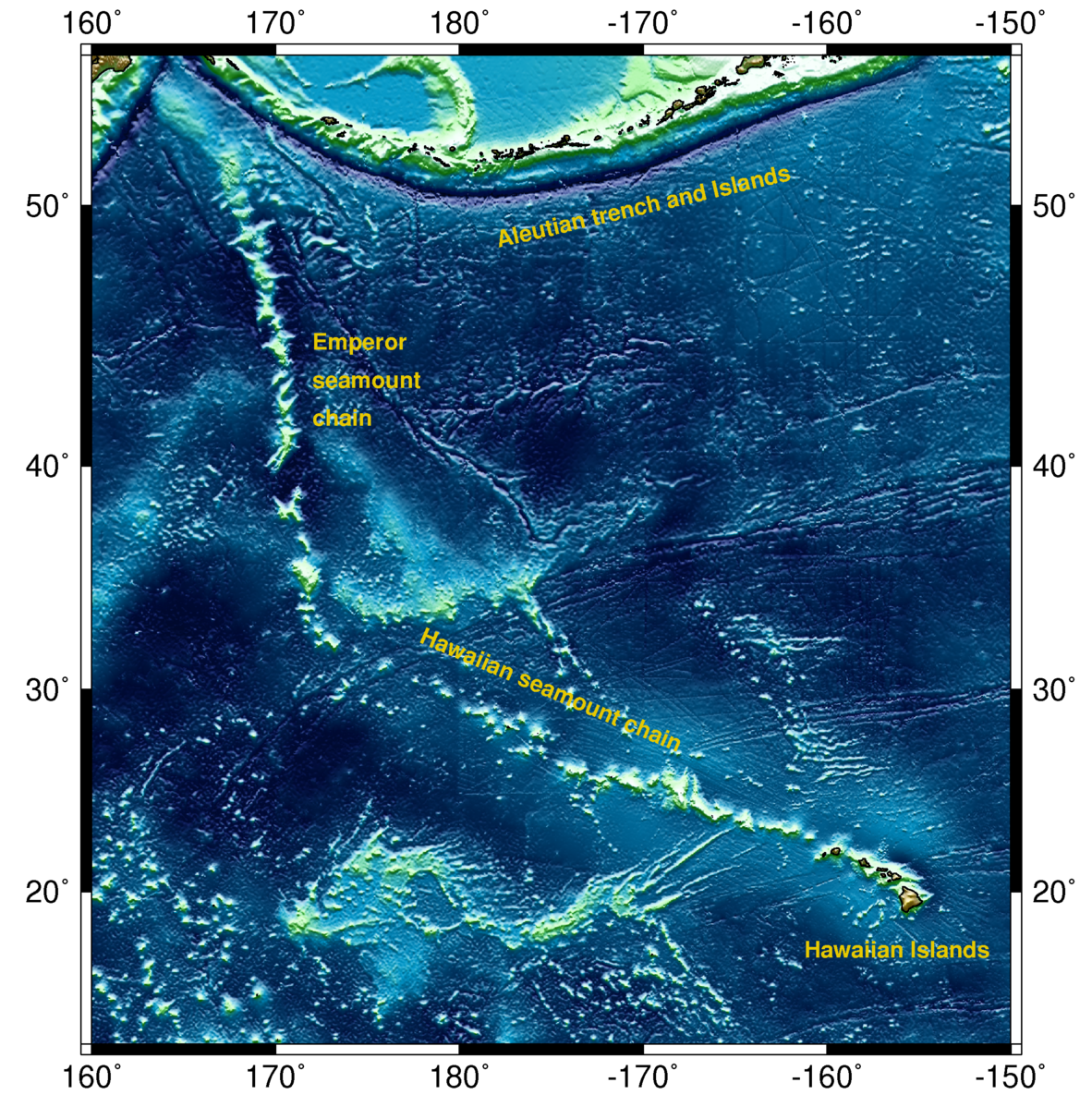
A zone of actively erupting volcanism connected to a chain of extinct volcanoes indicates intraplate volcanism located over a hotspot. These volcanic chains are created by the overriding oceanic plate slowly moving over a hotspot mantle plume. These chains are seen on the seafloor and continents and include volcanoes that have been inactive for millions of years. The Hawaiian Islands on the Pacific Oceanic plate are the active end of a long volcanic chain that extends from the northwest Pacific Ocean to the Emperor Seamounts, all the way to the subduction zone beneath the Kamchatka Peninsula. The overriding North American continental plate moved across a mantle plume hotspot for several million years, creating a chain of volcanic calderas that extends from Southwestern Idaho to the presently active Yellowstone caldera in Wyoming.
Two three-minute videos (below) illustrate hotspot volcanoes.
Volcano Features and Types
There are several different types of volcanoes based on their shape, eruption style, magmatic composition, and other aspects.
The figure shows the main features of a typical stratovolcano: 1) magma chamber, 2) upper layers of lithosphere, 3) the conduit or narrow pipe through which the lava erupts, 4) the base or edge of the volcano, 5) a sill of magma between layers of the volcano, 6) a diapir or feeder tube to the sill, 7) layers of tephra (ash) from previous eruptions, 8) & 9) layers of lava erupting from the vent and flowing down the sides of the volcano, 10) the crater at the top of the volcano, 11) layers of lava and tephra on 12), a parasitic cone. A parasitic cone is a small volcano located on the flank of a larger volcano such as Shastina on Mount Shasta. Kilauea sitting on the flank of Mauna Loa is not considered a parasitic cone because it has its own separate magma chamber [13]. 13) the vents of the parasite and the main volcano, 14) the rim of the crater, 15) clouds of ash blown into the sky by the eruption; this settles back onto the volcano and surrounding land.
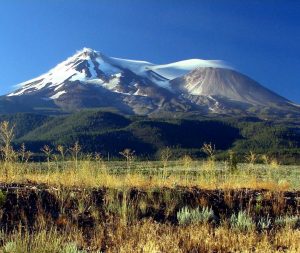
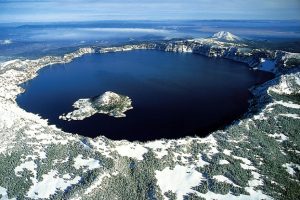
The largest craters are called calderas, such as the Crater Lake Caldera in Oregon. Many volcanic features are produced by viscosity, a basic property of lava. Viscosity is the resistance to flowing by a fluid. Low viscosity magma flows easily more like syrup, the basaltic volcanism that occurs in Hawaii on shield volcanoes. High viscosity means a thick and sticky magma, typically felsic or intermediate, that flows slowly, similar to toothpaste.
Shield Volcano
The largest volcanoes are shield volcanoes. They are characterized by broad low-angle flanks, small vents at the top, and mafic magma chambers. The name comes from the side view, which resembles a medieval warrior’s shield. They are typically associated with hotspots, mid-ocean ridges, or continental rifts with rising upper mantle material. The low-angle flanks are built up slowly from numerous low-viscosity basaltic lava flows that spread out over long distances. The basaltic lava erupts effusively, meaning the eruptions are small, localized, and predictable.
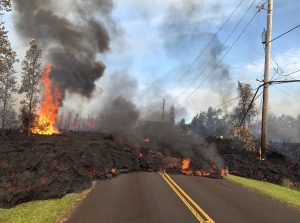
Typically, shield volcano eruptions are not much of a hazard to human life—although non-explosive eruptions of Kilauea (Hawaii) in 2018 produced uncharacteristically large lavas that damaged roads and structures. Mauna Loa (see USGS page) and Kilauea (see USGS page) in Hawaii are examples of shield volcanoes. Shield volcanoes are also found in Iceland, the Galapagos Islands, Northern California, Oregon, and the East African Rift [14].
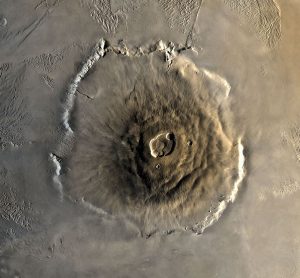
The largest volcanic edifice in the Solar System is Olympus Mons on Mars. This (possibly extinct) shield volcano covers an area the size of the state of Arizona. This may indicate the volcano erupted over a hotspot for millions of years, which means Mars had little, if any, plate tectonic activity. [15] [16].
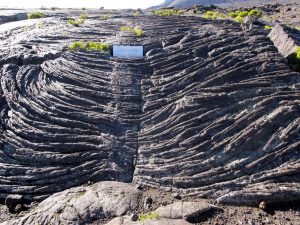
Basaltic lava forms special landforms based on magma temperature, composition, and content of dissolved gases and water vapor. The two main types of basaltic volcanic rock have Hawaiian names—pahoehoe and aa. Pahoehoe might come from low-viscosity lava that flows easily into ropey strands.
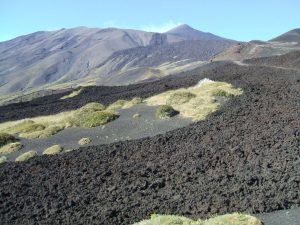
Aa (sometimes spelled a’a or ʻaʻā and pronounced “ah-ah”) is more viscous and has a crumbly blocky appearance [17]. The exact details of what forms the two types of flows are still up for debate. Felsic lavas have lower temperatures and more silica and thus are higher viscosity. These also form aa-style flows.
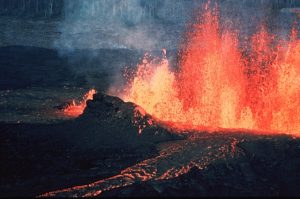
Low-viscosity, fast-flowing basaltic lava tends to harden on the outside into a tube and continue to flow internally. Once lava flow subsides, the empty outer shell may be left as a lava tube. Lava tubes, with or without collapsed roofs, make famous caves in Hawaii, Northern California, the Columbia River Basalt Plateau of Washington and Oregon, El Malpais National Monument in New Mexico, and Craters of the Moon National Monument in Idaho.
Fissures are cracks that commonly originate from shield-style eruptions. Lava emerging from fissures is typically mafic and very fluid. The 2018 Kiluaea eruption included fissures associated with the lava flows. Some fissures are caused by the volcanic seismic activity rather than lava flows. Some fissures are influenced by plate tectonics, such as the common fissures located parallel to the divergent boundary in Iceland.
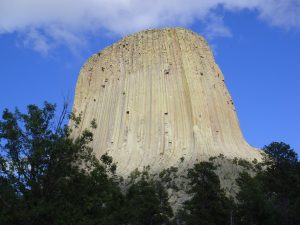
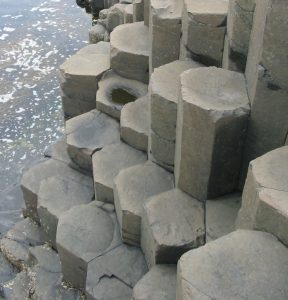
Cooling lava can contract into columns with semi-hexagonal cross sections called columnar jointing. This feature forms the famous Devils Tower in Wyoming, possibly an ancient volcanic vent from which the surrounding layers of lava and ash have been removed by erosion. Another well-known exposed example of columnar jointing is the Giant’s Causeway in Ireland.
Stratovolcano
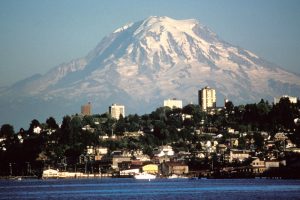
A stratovolcano, also called a composite cone volcano, has steep flanks, a symmetrical cone shape, a distinct crater, and rises prominently above the surrounding landscape. The term composite refers to the alternating layers of pyroclastic fragments like ash and bombs, and solidified lava flows of varying composition. Examples include Mount Rainier in Washington state and Mount Fuji in Japan.
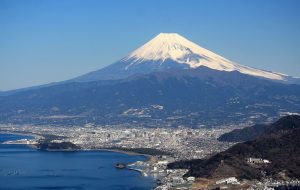
Stratovolcanoes usually have felsic to intermediate magma chambers, but can even produce mafic lavas. Stratovolcanoes have viscous lava flows and domes, punctuated by explosive eruptions. This produces volcanoes with steep flanks [14].
Lava Domes
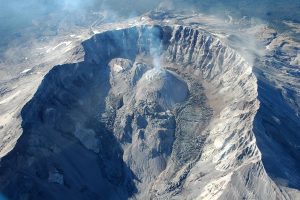
Lava domes are accumulations of silica-rich volcanic rock, such as rhyolite and obsidian. Too viscous to flow easily, the felsic lava tends to pile up near the vent in blocky masses. Lava domes often form in a vent within the collapsed crater of a stratovolcano and grow by internal expansion. As the dome expands, the outer surface cools, hardens, and shatters, and spills loose fragments down the sides. Mount Saint Helens has a good example of a lava dome inside of a collapsed stratovolcano crater. Examples of stand-alone lava domes are Chaiten in Chile and Mammoth Mountain in California [18][14].
Caldera
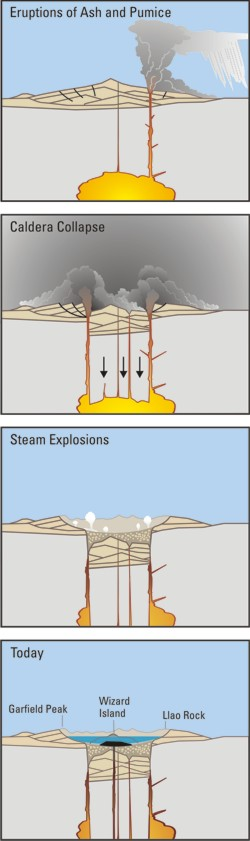
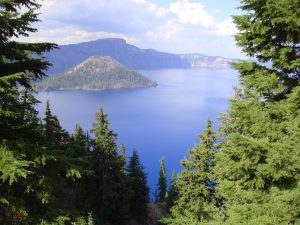
Calderas are steep-walled, basin-shaped depressions formed by the collapse of a volcanic edifice into an empty magma chamber. Calderas are generally very large, with diameters of up to 25 km (15.5 mi). The term caldera specifically refers to a volcanic vent but it is frequently used to describe a volcano type. Caldera volcanoes are typically formed by eruptions of high-viscosity felsic lava having high volatiles content.
Crater Lake, Yellowstone, and the Long Valley Caldera are good examples of this type of volcanism. The caldera at Crater Lake National Park in Oregon was created about 6,800 years ago when Mount Mazama, a composite volcano, erupted in a huge explosive blast. The volcano ejected large amounts of volcanic ash and rapidly drained the magma chamber, causing the top to collapse into a large depression that later filled with water. Wizard Island in the middle of the lake is a later resurgent lava dome that formed within the caldera basin [14].
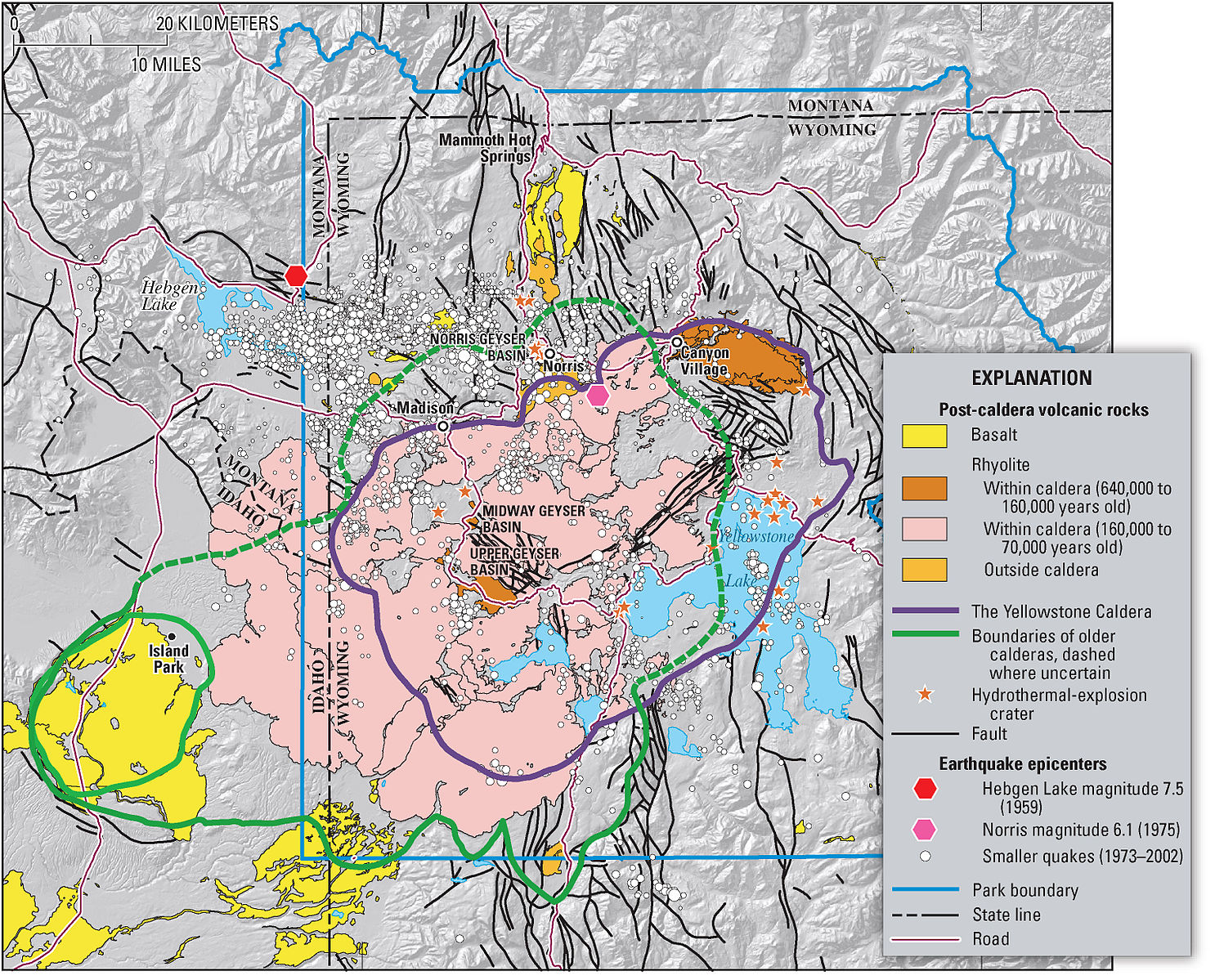
The Yellowstone volcanic system erupted three times in the recent geologic past—2.1, 1.3, and 0.64 million years ago—leaving behind three caldera basins. Each eruption created large rhyolite lava flows as well as pyroclastic flows that solidified into tuff formations. These extra-large eruptions rapidly emptied the magma chamber, causing the roof to collapse and form a caldera. The youngest of the three calderas contains most of Yellowstone National Park, as well as two resurgent lava domes. The calderas are difficult to see today due to the amount of time since their eruptions and subsequent erosion and glaciation.
Yellowstone volcanism started about 17-million years ago as a hotspot under the North American lithospheric plate near the Oregon/Nevada border. As the plate moved to the southwest over the stationary hotspot, it left behind a track of past volcanic activities. Idaho’s Snake River Plain was created from volcanism that produced a series of calderas and lava flows. The plate eventually arrived at its current location in northwestern Wyoming, where hotspot volcanism formed the Yellowstone calderas [19].
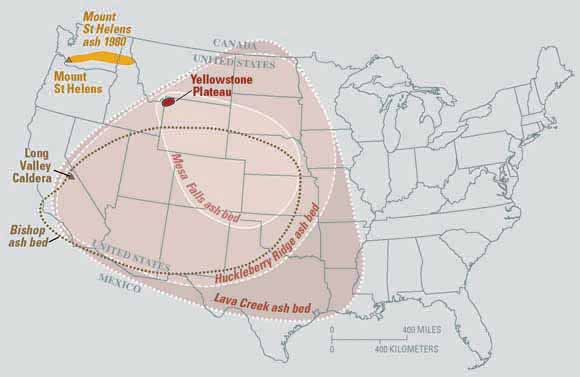
The Long Valley Caldera near Mammoth, California, is the result of a large volcanic eruption that occurred 760,000 years ago. The explosive eruption dumped enormous amounts of ash across the United States, in a manner similar to the Yellowstone eruptions. The Bishop Tuff deposit near Bishop, California, is made of ash from this eruption. The current caldera basin is 17 km by 32 km (10 mi by 20 mi), large enough to contain the town of Mammoth Lakes, major ski resort, airport, major highway, resurgent dome, and several hot springs [20].
Cinder Cone
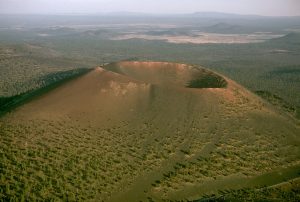
Cinder cones are small volcanoes with steep sides and made of pyroclastic fragments that have been ejected from a pronounced central vent. The small fragments are called cinders and the largest are volcanic bombs. The eruptions are usually short-lived events, typically consisting of mafic lavas with a high content of volatiles. Hot lava is ejected into the air, cooling and solidifying into fragments that accumulate on the flank of the volcano. Cinder cones are found throughout western North America [14].
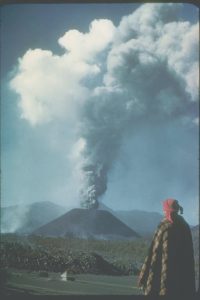
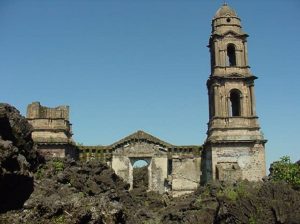
A recent and striking example of a cinder cone is the eruption near the village of Parícutin, Mexico that started in 1943 [21]. The cinder cone started explosively shooting cinders out of the vent in the middle of a farmer’s field. The volcanism quickly built up the cone to a height of over 90 m (300 ft) within a week and 365 m (1,200 ft) within the first 8 months. After the initial explosive eruption of gases and cinders, basaltic lava poured out from the base of the cone. This is a common order of events for cinder cones: violent eruption, cone, and crater formation, low-viscosity lava flow from the base. The cinder cone is not strong enough to support a column of lava rising to the top of the crater, so the lava breaks through and emerges near the bottom of the volcano. During nine years of eruption activity, the ashfall covered about 260 km2 (100 mi2) and destroyed the nearby town of San Juan [14].
Flood Basalts
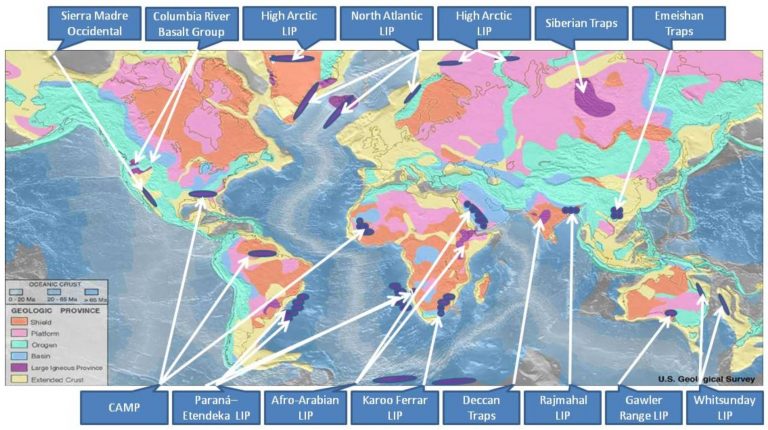
A rare volcanic eruption type, unobserved in modern times, is the flood basalt. Flood basalts are some of the largest and lowest viscosity types of eruptions known. They are not known from any eruption in human history, so the exact mechanisms of eruption are still mysterious. Some famous examples include the Columbia River Flood Basalts in Washington, Oregon, and Idaho, the Deccan Traps, which cover about 1/3 of the country of India, and the Siberian Traps, which may have been involved in the Earth’s largest mass extinction (see chapter 8).
Carbonatites
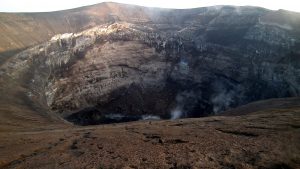
Arguably the most unusual volcanic activity is carbonatite eruptions. Only one actively erupting carbonatite volcano exists on Earth today, Ol Doinyo Lengai, in the East African Rift Zone of Tanzania. While all other volcanism on Earth originates from silicate-based magma, carbonatites are a product of carbonate-based magma and produce volcanic rocks containing greater than 50% carbonate minerals. Carbonatite lavas are very low viscosity and relatively cold for lava. The erupting lava is black and solidifies to brown/grey rock that eventually turns white. These rocks are occasionally found in the geologic record and require special study to distinguish them from metamorphic marbles (see Chapter 6). They are mostly associated with continental rifting [22].
Igneous rock types and related volcano types. Mid-ocean ridges and shield volcanoes represent more mafic compositions, and strato (composite) volcanoes generally represent a more intermediate or felsic composition and a convergent plate tectonic boundary. Note that there are exceptions to this generalized layout of volcano types and igneous rock composition.
Volcanic Hazards and Monitoring
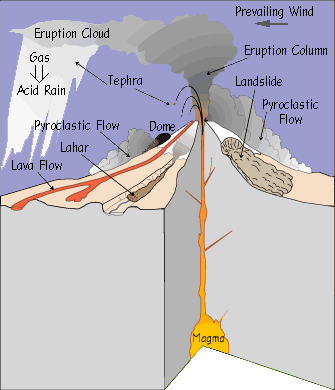
While the most obvious volcanic hazard is lava, the dangers posed by volcanoes go far beyond lava flows. For example, on May 18, 1980, Mount Saint Helens (Washington, United States) erupted with an explosion and landslide that removed the upper 400 m (1,300 ft) of the mountain. The initial explosion was immediately followed by a lateral blast, which produced a pyroclastic flow that covered nearly 600 km2 (230 mi2) of the forest with hot ash and debris [23]. The pyroclastic flow moved at speeds of 80-130 kph (50-80 mph), flattening trees and ejecting clouds of ash into the air. The USGS video provides an account of this explosive eruption that killed 57 people [24].
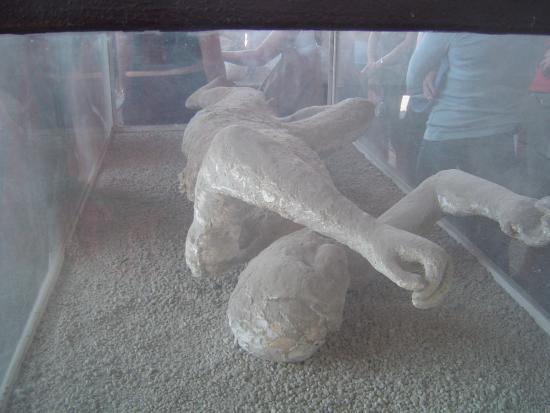
In 79 AD, Mount Vesuvius, located near Naples, Italy, violently erupted sending a pyroclastic flow over the Roman countryside, including the cities of Herculaneum and Pompeii. The buried towns were discovered in an archeological expedition in the 18th century [25]. Pompeii famously contains the remains (casts) of people suffocated by ash and covered by 10 feet (3 m) of ash, pumice lapilli, and collapsed roofs [26].
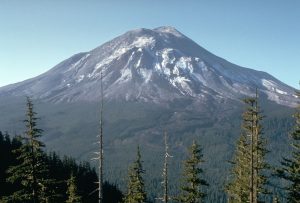
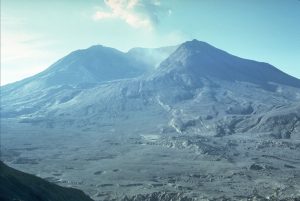
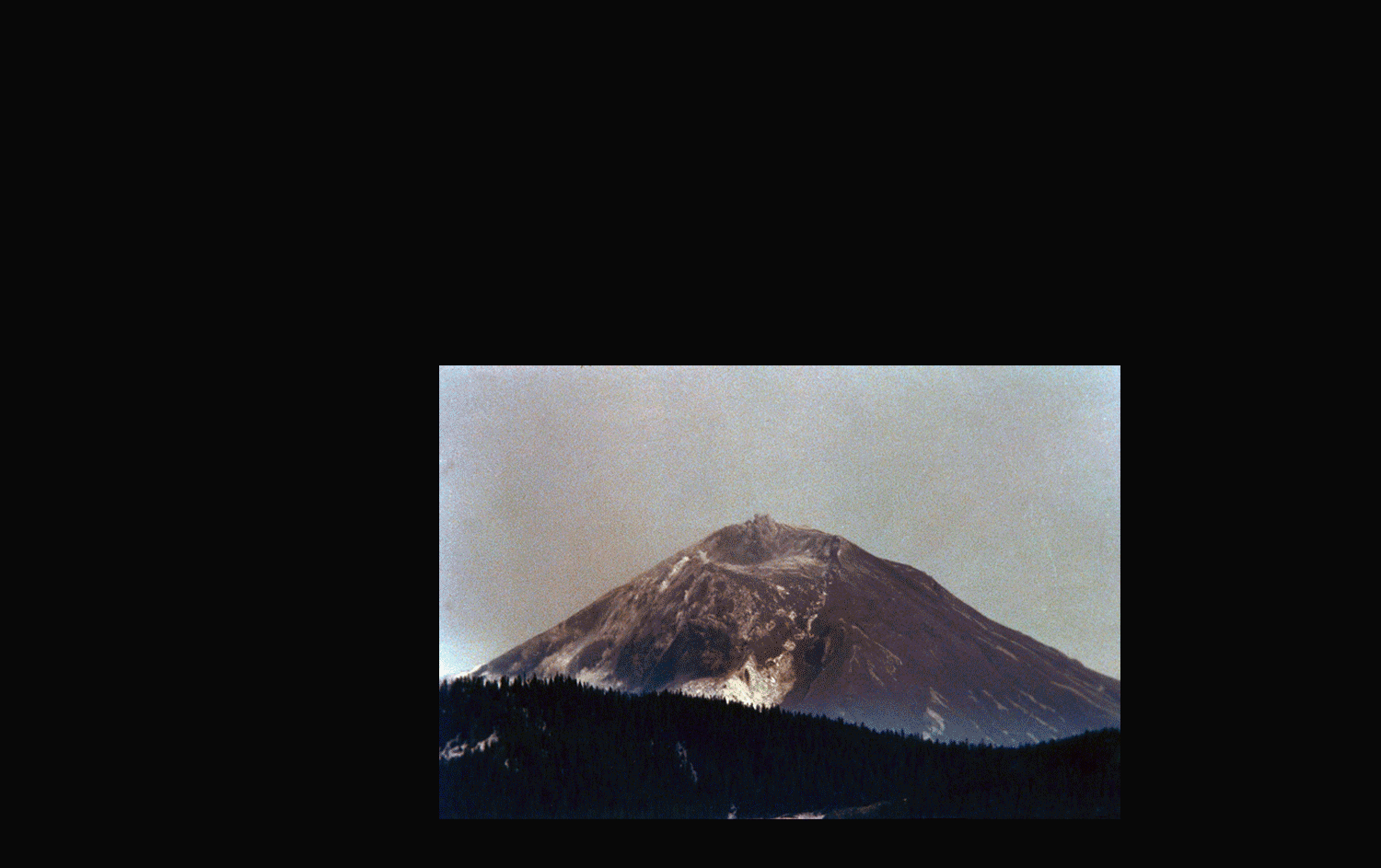
Pyroclastic Flows
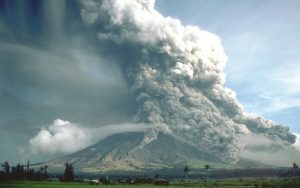
The most dangerous volcanic hazard are pyroclastic flows (video). These flows are a mix of lava blocks, pumice, ash, and hot gases between 200°C-700°C (400°F-1,300°F). The turbulent cloud of ash and gas races down the steep flanks at high speeds up to 193 kph (120 mph) into the valleys around composite volcanoes [24]. Most explosive, silica-rich, high viscosity magma volcanoes such as composite cones usually have pyroclastic flows. The rock tuff and welded tuff is often formed from these pyroclastic flows.
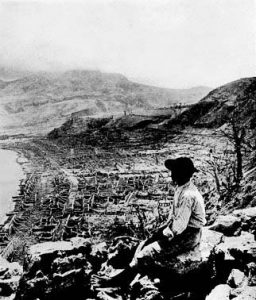
There are numerous examples of deadly pyroclastic flows. In 2014, the Mount Ontake pyroclastic flow in Japan killed 47 people. The flow was caused by magma heating groundwater into steam, which then rapidly ejected with ash and volcanic bombs. Some were killed by inhalation of toxic gases and hot ash, while others were struck by volcanic bombs [27]. Two short videos below document an eye-witness video of pyroclastic flows. In the early 1990s, Mount Unzen erupted several times with pyroclastic flows. The pyroclastic flow shown in this famous short video killed 41 people. In 1902, on the Caribbean Island Martinique, Mount Pelee erupted with a violent pyroclastic flow that destroyed the entire town of St. Pierre and killing 28,000 people in moments [28].
Landslides and Landslide-Generated Tsunamis
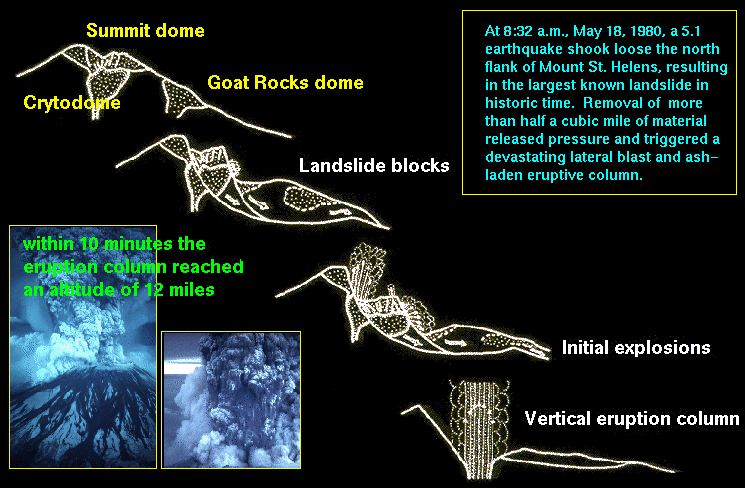
The steep and unstable flanks of a volcano can lead to slope failure and dangerous landslides. These landslides can be triggered by magma movement, explosive eruptions, large earthquakes, and/or heavy rainfall. During the 1980 Mount St. Helens eruption, the entire north flank of the volcano collapsed and released a huge landslide that moved at speeds of 160-290 kph (100-180 mph).
If enough landslide material reaches the ocean, it may cause a tsunami. In 1792, a landslide caused by the Mount Unzen eruption reached the Ariaka Sea, generating a tsunami that killed 15,000 people (see USGS page). When Mount Krakatau in Indonesia erupted in 1883, it generated ocean waves that towered 40 m (131 ft) above sea level. The tsunami killed 36,000 people and destroyed 165 villages [24].
Tephra
Volcanoes, especially composite volcanoes, eject large amounts of tephra (ejected rock materials), most notably ash (tephra fragments less than 0.08 inches [2 mm]). Larger tephra is heavier and falls closer to the vent. Larger blocks and bombs pose hazards to those close to the eruption such as at the 2014 Mount Ontake disaster in Japan discussed earlier.
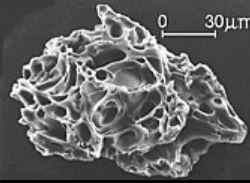
Hot ash poses an immediate danger to people, animals, plants, machines, roads, and buildings located close to the eruption. Ash is fine-grained (< 2mm) and can travel airborne long distances away from the eruption site. Heavy accumulations of ash can cause buildings to collapse. In people, it may cause respiratory issues like silicosis. Ash is destructive to aircraft and automobile engines, which can disrupt transportation and shipping services [24]. In 2010, the Eyjafjallajökull volcano in Iceland emitted a large ash cloud into the upper atmosphere, causing the largest air-travel disruption in northern Europe since World War II. No one was injured, but the service disruption was estimated to have cost the world economy billions of dollars [29].
Volcanic Ashes
As magma rises to the surface, the confining pressure decreases and allows dissolved gases to escape into the atmosphere. Even volcanoes that are not actively erupting may emit hazardous gases, such as carbon dioxide (CO2), sulfur dioxide (SO2), hydrogen sulfide (H2S), and hydrogen halides (HF, HCl, or HBr).
Carbon dioxide tends to sink and accumulate in depressions and basins. In volcanic areas known to emit carbon dioxide, low-lying areas may trap hazardous concentrations of this colorless and odorless gas. The Mammoth Mountain Ski Resort in California, is located within the Long Valley Caldera, is one such area of carbon dioxide-producing volcanism. In 2006, three ski patrol members died of suffocation caused by carbon dioxide after falling into a snow depression near a fumarole (info).
In rare cases, volcanism may create a sudden emission of gases without warning. Limnic eruptions (limne is Greek for lake), occur in crater lakes associated with active volcanism. The water in these lakes is supercharged with high concentrations of dissolved gases. If the water is physically jolted by a landslide or earthquake, it may trigger an immediate and massive release of gases out of solution. An analogous example would be what happens to a vigorously shaken bottle of carbonated soda when the cap is opened. An infamous limnic eruption occurred in 1986 at Lake Nyos, Cameroon. Almost 2,000 people were killed by a massive release of carbon dioxide [24].
Lahars
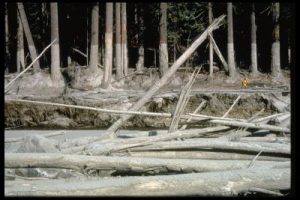
Lahar is an Indonesian word and is used to describe a volcanic mudflow that forms from rapidly melting snow or glaciers. Lahars are slurries resembling wet concrete and consist of water, ash, rock fragments, and other debris. These mudflows flow down the flanks of volcanoes or mountains covered with freshly-erupted ash and on steep slopes can reach speeds of up to 80 kph (50 mph).
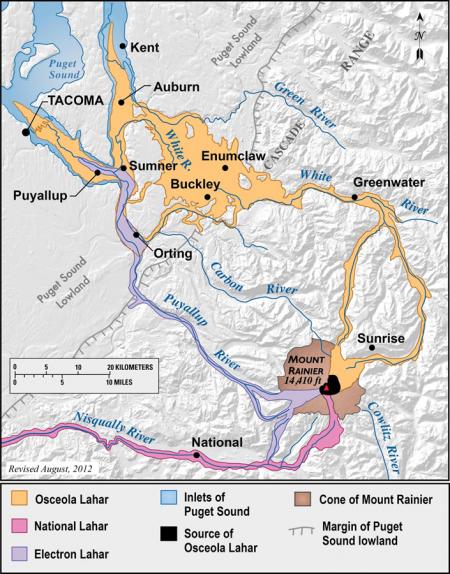
Several major cities, including Tacoma, are located on prehistoric lahar flows that extend for many kilometers across the flood plains surrounding Mount Rainier in Washington (see map). A map of Mount Baker in Oregon shows a similar potential hazard for lahar flows (see map) [24]. A tragic scenario played out recently, in 1985, when a lahar from the Nevado del Ruiz volcano in Colombia buried the town of Armero and killed an estimated 23,000 people.
Monitoring
Geologists use various instruments to detect changes or indications that an eruption is imminent [30][31]. The three videos show different types of volcanic monitoring used to predict eruptions 1) earthquake activity, 2) increases in gas emission, and 3) changes in land surface orientation and elevation.
One video shows how monitoring earthquake frequency, especially special vibrational earthquakes called harmonic tremors, can detect magma movement and possible eruption. Another video shows how gas monitoring may be used to predict an eruption. A rapid increase of gas emission may indicate magma that is actively rising to surface and releasing dissolved gases out of solution, and that an eruption is imminent. The last video shows how a GPS unit and tiltmeter can detect land surface changes, indicating the magma is moving underneath it.