8.1: Introduction to Soil Health
- Page ID
- 14465
\( \newcommand{\vecs}[1]{\overset { \scriptstyle \rightharpoonup} {\mathbf{#1}} } \)
\( \newcommand{\vecd}[1]{\overset{-\!-\!\rightharpoonup}{\vphantom{a}\smash {#1}}} \)
\( \newcommand{\id}{\mathrm{id}}\) \( \newcommand{\Span}{\mathrm{span}}\)
( \newcommand{\kernel}{\mathrm{null}\,}\) \( \newcommand{\range}{\mathrm{range}\,}\)
\( \newcommand{\RealPart}{\mathrm{Re}}\) \( \newcommand{\ImaginaryPart}{\mathrm{Im}}\)
\( \newcommand{\Argument}{\mathrm{Arg}}\) \( \newcommand{\norm}[1]{\| #1 \|}\)
\( \newcommand{\inner}[2]{\langle #1, #2 \rangle}\)
\( \newcommand{\Span}{\mathrm{span}}\)
\( \newcommand{\id}{\mathrm{id}}\)
\( \newcommand{\Span}{\mathrm{span}}\)
\( \newcommand{\kernel}{\mathrm{null}\,}\)
\( \newcommand{\range}{\mathrm{range}\,}\)
\( \newcommand{\RealPart}{\mathrm{Re}}\)
\( \newcommand{\ImaginaryPart}{\mathrm{Im}}\)
\( \newcommand{\Argument}{\mathrm{Arg}}\)
\( \newcommand{\norm}[1]{\| #1 \|}\)
\( \newcommand{\inner}[2]{\langle #1, #2 \rangle}\)
\( \newcommand{\Span}{\mathrm{span}}\) \( \newcommand{\AA}{\unicode[.8,0]{x212B}}\)
\( \newcommand{\vectorA}[1]{\vec{#1}} % arrow\)
\( \newcommand{\vectorAt}[1]{\vec{\text{#1}}} % arrow\)
\( \newcommand{\vectorB}[1]{\overset { \scriptstyle \rightharpoonup} {\mathbf{#1}} } \)
\( \newcommand{\vectorC}[1]{\textbf{#1}} \)
\( \newcommand{\vectorD}[1]{\overrightarrow{#1}} \)
\( \newcommand{\vectorDt}[1]{\overrightarrow{\text{#1}}} \)
\( \newcommand{\vectE}[1]{\overset{-\!-\!\rightharpoonup}{\vphantom{a}\smash{\mathbf {#1}}}} \)
\( \newcommand{\vecs}[1]{\overset { \scriptstyle \rightharpoonup} {\mathbf{#1}} } \)
\( \newcommand{\vecd}[1]{\overset{-\!-\!\rightharpoonup}{\vphantom{a}\smash {#1}}} \)
\(\newcommand{\avec}{\mathbf a}\) \(\newcommand{\bvec}{\mathbf b}\) \(\newcommand{\cvec}{\mathbf c}\) \(\newcommand{\dvec}{\mathbf d}\) \(\newcommand{\dtil}{\widetilde{\mathbf d}}\) \(\newcommand{\evec}{\mathbf e}\) \(\newcommand{\fvec}{\mathbf f}\) \(\newcommand{\nvec}{\mathbf n}\) \(\newcommand{\pvec}{\mathbf p}\) \(\newcommand{\qvec}{\mathbf q}\) \(\newcommand{\svec}{\mathbf s}\) \(\newcommand{\tvec}{\mathbf t}\) \(\newcommand{\uvec}{\mathbf u}\) \(\newcommand{\vvec}{\mathbf v}\) \(\newcommand{\wvec}{\mathbf w}\) \(\newcommand{\xvec}{\mathbf x}\) \(\newcommand{\yvec}{\mathbf y}\) \(\newcommand{\zvec}{\mathbf z}\) \(\newcommand{\rvec}{\mathbf r}\) \(\newcommand{\mvec}{\mathbf m}\) \(\newcommand{\zerovec}{\mathbf 0}\) \(\newcommand{\onevec}{\mathbf 1}\) \(\newcommand{\real}{\mathbb R}\) \(\newcommand{\twovec}[2]{\left[\begin{array}{r}#1 \\ #2 \end{array}\right]}\) \(\newcommand{\ctwovec}[2]{\left[\begin{array}{c}#1 \\ #2 \end{array}\right]}\) \(\newcommand{\threevec}[3]{\left[\begin{array}{r}#1 \\ #2 \\ #3 \end{array}\right]}\) \(\newcommand{\cthreevec}[3]{\left[\begin{array}{c}#1 \\ #2 \\ #3 \end{array}\right]}\) \(\newcommand{\fourvec}[4]{\left[\begin{array}{r}#1 \\ #2 \\ #3 \\ #4 \end{array}\right]}\) \(\newcommand{\cfourvec}[4]{\left[\begin{array}{c}#1 \\ #2 \\ #3 \\ #4 \end{array}\right]}\) \(\newcommand{\fivevec}[5]{\left[\begin{array}{r}#1 \\ #2 \\ #3 \\ #4 \\ #5 \\ \end{array}\right]}\) \(\newcommand{\cfivevec}[5]{\left[\begin{array}{c}#1 \\ #2 \\ #3 \\ #4 \\ #5 \\ \end{array}\right]}\) \(\newcommand{\mattwo}[4]{\left[\begin{array}{rr}#1 \amp #2 \\ #3 \amp #4 \\ \end{array}\right]}\) \(\newcommand{\laspan}[1]{\text{Span}\{#1\}}\) \(\newcommand{\bcal}{\cal B}\) \(\newcommand{\ccal}{\cal C}\) \(\newcommand{\scal}{\cal S}\) \(\newcommand{\wcal}{\cal W}\) \(\newcommand{\ecal}{\cal E}\) \(\newcommand{\coords}[2]{\left\{#1\right\}_{#2}}\) \(\newcommand{\gray}[1]{\color{gray}{#1}}\) \(\newcommand{\lgray}[1]{\color{lightgray}{#1}}\) \(\newcommand{\rank}{\operatorname{rank}}\) \(\newcommand{\row}{\text{Row}}\) \(\newcommand{\col}{\text{Col}}\) \(\renewcommand{\row}{\text{Row}}\) \(\newcommand{\nul}{\text{Nul}}\) \(\newcommand{\var}{\text{Var}}\) \(\newcommand{\corr}{\text{corr}}\) \(\newcommand{\len}[1]{\left|#1\right|}\) \(\newcommand{\bbar}{\overline{\bvec}}\) \(\newcommand{\bhat}{\widehat{\bvec}}\) \(\newcommand{\bperp}{\bvec^\perp}\) \(\newcommand{\xhat}{\widehat{\xvec}}\) \(\newcommand{\vhat}{\widehat{\vvec}}\) \(\newcommand{\uhat}{\widehat{\uvec}}\) \(\newcommand{\what}{\widehat{\wvec}}\) \(\newcommand{\Sighat}{\widehat{\Sigma}}\) \(\newcommand{\lt}{<}\) \(\newcommand{\gt}{>}\) \(\newcommand{\amp}{&}\) \(\definecolor{fillinmathshade}{gray}{0.9}\)Introduction to Soil Health
(Adapted from NRCS, 2017)
Soil health is also commonly referred to as, soil quality. As defined by the Natural Resource Conservation Service, soil health is “the continued capacity of soil to function as a vital living ecosystem that sustains plants, animals, and humans.” This concept identifies the fact that only “living” things can have health, thereby stressing the concept that a soil is a living ecosystem. As with all living entities, including living ecosystems, the only way to maintain life is through proper management.
Soil is not simply an inert growing medium, but rather it is comprised of billions of biological organisms, ranging from microscopic to macroscopic in size. Together these organisms help to maintain the life of the soil, as well as many physical, chemical and biological factors. Therefore, soil is an ecosystem which can provide the following capabilities: (NRCS, 2017)
- Regulating water - Soil helps control where rain, snowmelt, and irrigation water goes. Water and dissolved solutes flow over the land or into and through the soil.
- Sustaining plant and animal life - The diversity and productivity of living things depends on soil.
- Filtering and buffering potential pol utants - The minerals and microbes in soil are responsible for filtering, buffering, degrading, immobilizing, and detoxifying organic and inorganic materials, including industrial and municipal by-products and atmospheric deposits.
- Cycling nutrients - Carbon, nitrogen, phosphorus, and many other nutrients are stored, transformed, and cycled in the soil.
- Physical stability and support - Soil structure provides a medium for plant roots. Soils also provide support for human structures and protection for archeological treasures.
The capabilities of a soil are determined by both inherent and dynamic properties/qualities within the soil. Inherent soil quality is, “a soil’s natural ability to function.” An example of an inherent soil property/quality is how a sandy texture soil drains faster than a clayey texture soil, or how a deep soil has more space for root growth compared to a soil with bedrock at or near the surface. Essentially the idea behind inherent properties/qualities is that these characteristics do not change easily. On the contrary, dynamic soil quality is, “how soil changes depending on how it is managed.” Management decisions and efforts can affect factors such as the amount of soil organic matter, soil structure, and water and nutrient holding capacity. Thus, dynamic soil quality is directly affected by inherent soil quality.
One major goal of soil health is to manage the soil in a way that enhances or improves soil function. It is important to understand that soils wil respond differently to management depending on inherent soil properties, and so how these properties vary across the surrounding landscape should be taken into consideration. Another core goal of soil health is to assess and manage soil so that it functions optimal y for current and future use. Monitoring changes in soil health is critical for determining sustainable soil management and practices.
Aggregate Stability
A soil with strong aggregates generally has adequate biological activity, organic matter, and nutrient cycling present. When soil aggregates are weak, it may be a sign of degradation.
Leaving soil vulnerable to greater risk of wind and water erosion, and impeding infiltration and root growth. Aggregation is especially important for survival of biological soil communities and will affect the amount of pore space between the soil particles, therefore also impacting bulk density, CEC, and many other dynamic soil properties.
Soil particles form aggregates as a product of cementing agents in the soil including clay content, adsorbed cations such as calcium and magnesium, and iron oxide content. Expansion and contraction of clay particles as they become moist and then dry can shift and crack the soil mass and create aggregates or break them apart. Calcium, magnesium, iron, and aluminum stabilize aggregates via organic matter sorption. In contrast, aggregate stability decreases with increasing amounts of exchangeable sodium: dispersion is promoted when too many sodium ions accumulate between soil particles.
Tilling a soil can both promote and destroy soil aggregates. If the soil is at an appropriate moisture level, tillage can break large clods into natural aggregates, creating a loose, porous condition that is conducive to the growth of young roots and seedlings, as well as incorporating organic amendments into the soil and killing weeds. However, over many years, tilling practices can increase the oxidative loss of soil organic matter, weakening soil aggregates. If tillage is carried out when soil is too wet, soil aggregates can be crushed or smeared, resulting decreased macro porosity and creation of ponding conditions.
One method to determine if a soil has stable aggregates is to perform a slaking test, which is recommended by the USDA Natural Resources Conservation Service.
NUTRIENT CYCLING
There are several plant-available nutrients that are important to the overall health of a soil. The three major nutrients of importance are N, P, and K. Nutrients occur naturally in soils in mineral form, through biological inputs, atmospheric deposition, and the application of fertilizers. Nutrients are lost from systems through processes like runoff, water solubility, plant uptake and leaching. When managing a soil for plant production, it is important to understand nutrient cycles, availability, and potential for loss.
The Nitrogen Cycle
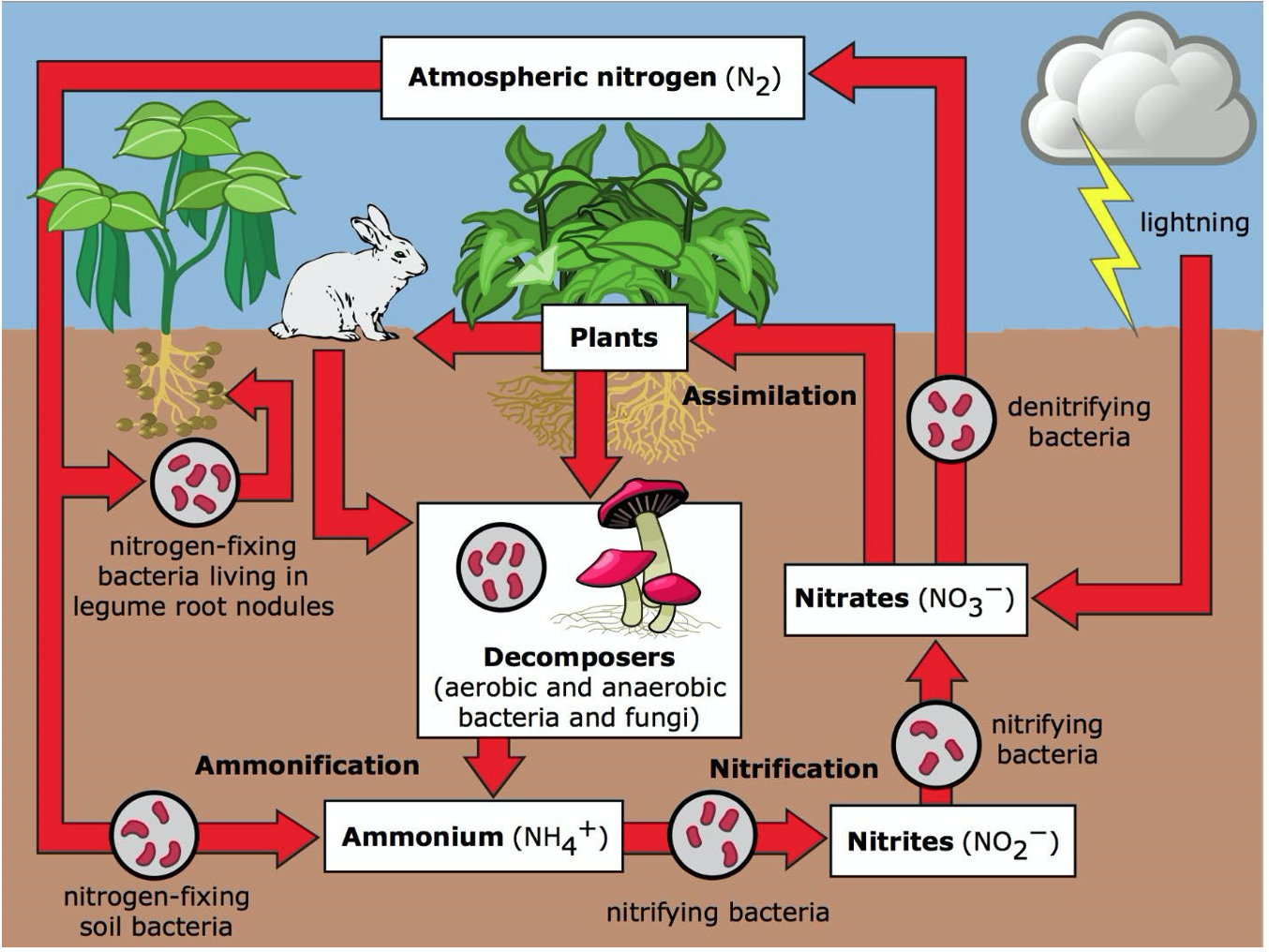
Nitrification is the oxidation of reduced forms of N, typically ammonium (\(\ce{NH4+}\)), via nitrite (\(\ce{NO2}\)), to nitrate (\(\ce{NO3^{-}}\)). The process begins with autotrophic and chemolithotrophic bacteria oxidizing ammonium generated by organic matter mineralization and fertilizer addition, producing nitrate and acid, leading to soil acidification. The process of nitrification determines the relative amounts of different inorganic N sources available for plant and crop growth and is responsible for significant loss of added N fertilizer.
Denitrification involves the reduction of nitrate to nitrite, nitric oxide, nitrous oxide and nitrogen gas. This process is catalyzed by bacteria, archaea and fungi, and is essential for returning N to the atmosphere.
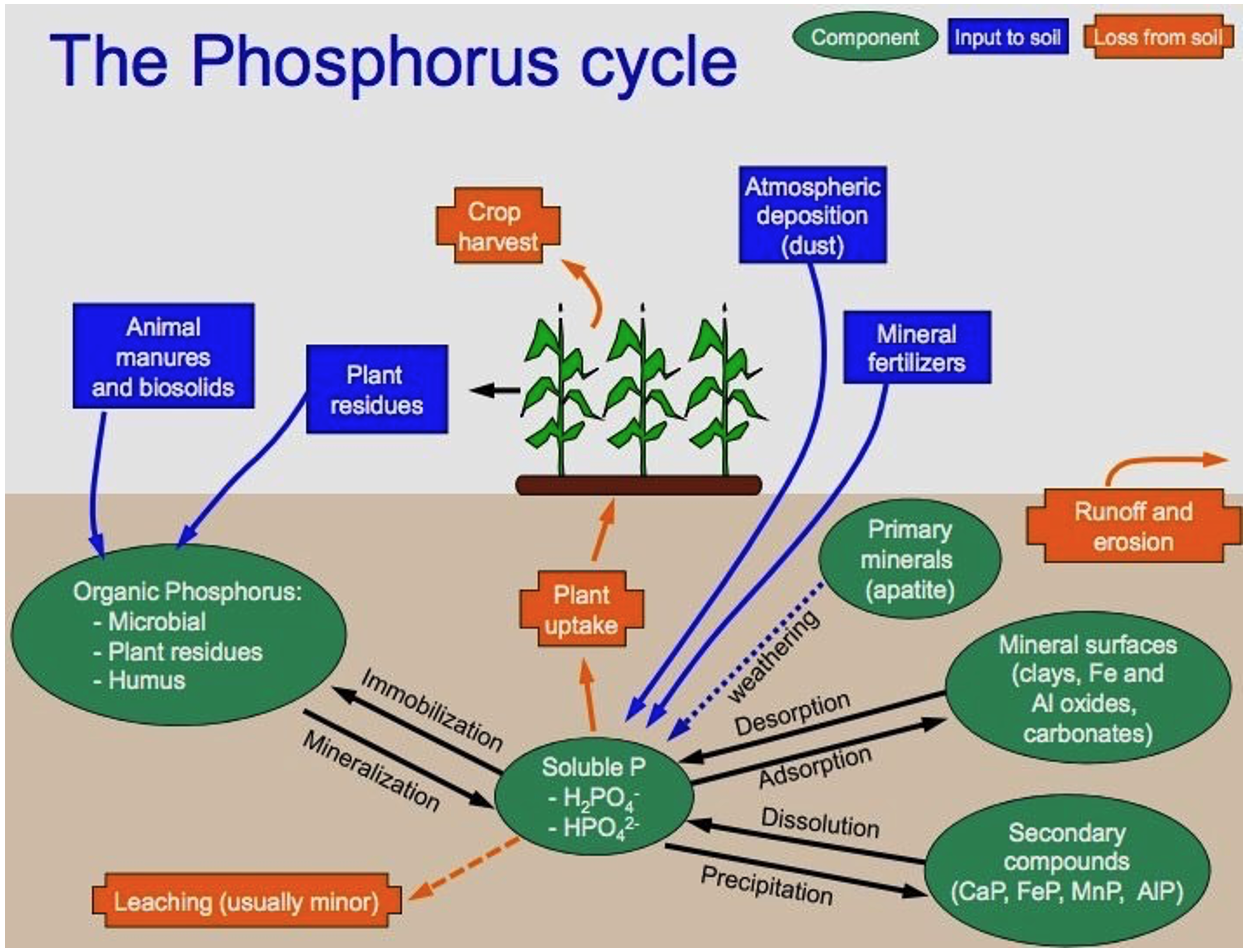
Compared to other macronutrients, like S and Ca, the concentration of phosphorus in the soil solution is very low, generally ranging from 0.001 mg/L in infertile soil to 1 mg/L in heavily fertilized soils. Plant roots absorb P dissolved in soil solution, mainly as phosphate ions (HPO42- and H2PO4 ), determined by the soil pH. In strongly acid soils (pH 4 5.5), H2PO42- dominates, while alkaline soils contain mostly HPO42-. Phosphorus is lost from the soil by plant removal, erosion of P-carrying soil particles, P dissolved in surface runoff water and leaching to groundwater. Additions of P to the soil from the atmosphere are smal but may balance losses in undisturbed ecosystems. For optimal crop production, input from fertilizer to exceed the removal in crop harvest may be required. Phosphorus held in organic forms can be mineralized and immobilized by the same general processes that release N and C from SOM: immobilization and mineralization. 1 These processes are influenced by the same factors that control general decomposition of SOM: temperature, moisture, and tillage.
1 Net immobilization of soluble P is likely to occur if residues added to the soil have a C/P ratio that is greater than 300:1, while net mineralization is likely if the ratio is below 200:1.
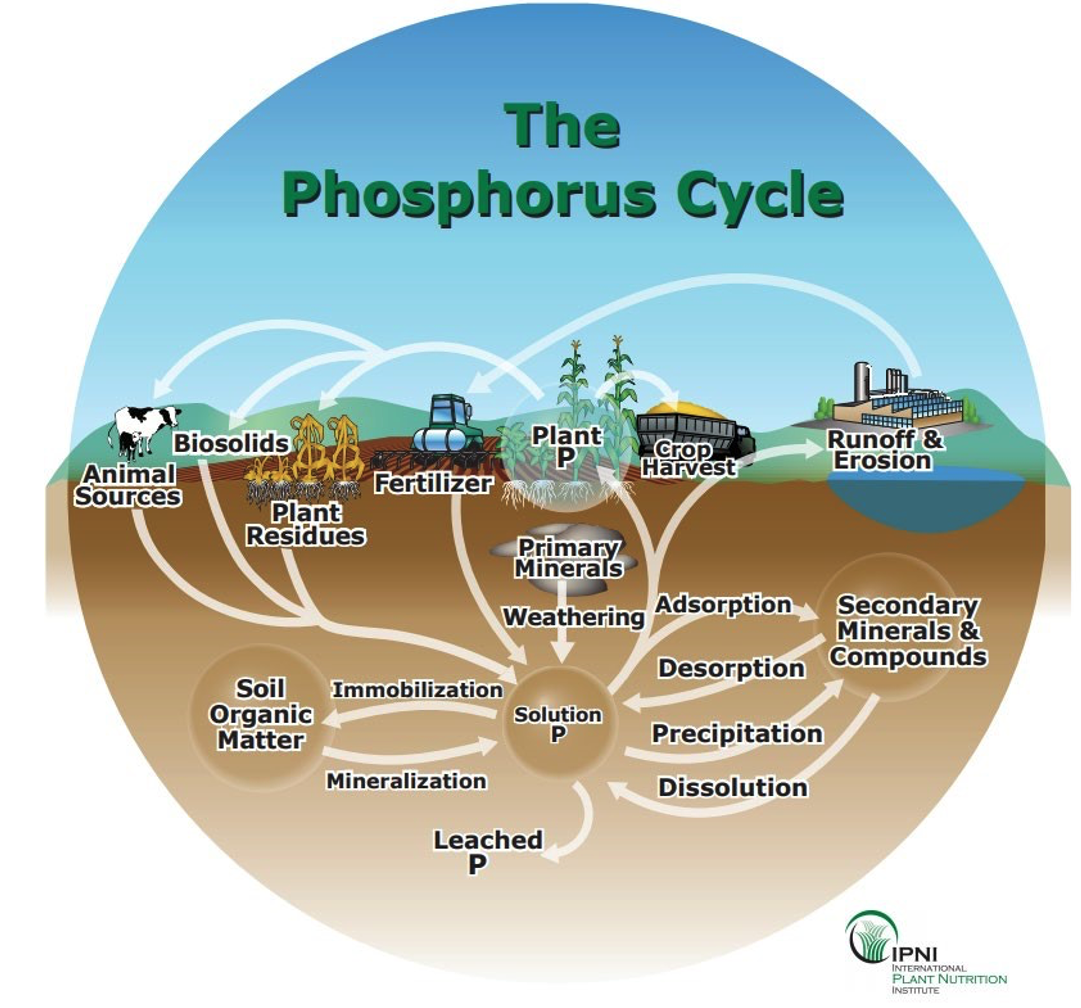
In contrast to phosphorus, potassium is found in comparatively high levels in most mineral soils, yet the quantity of K held in an easily exchangeable condition is often very small. However, over time K can be released to exchangeable and dissolved forms in the soil solution and can be quickly taken up by plants.
Potassium is readily lost by leaching, which can be reduced by increasing the cation exchange capacity (CEC) of the soil.2 Liming an acidic soil to raise pH can reduce the leaching losses as wel . Plants take up very large amounts of K so biomass removal is another source of K loss from soils. This situation is increased by luxury consumption, or the tendency of plants to take up soluble potassium in excess if sufficiently large quantities are present.
2 CEC is the attraction of positively charged potassium ions to the negatively charged cation exchange sites on clay and organic matter.
Forms of potassium in soils, as shown in the graphic above, include K in primary mineral structure (unavailable to plant uptake), nonexchangeable K in secondary minerals & compounds (slowly available), and exchangeable K+ on soil colloids and K2O soluble in water (readily available, only 1-2% of total soil potassium).
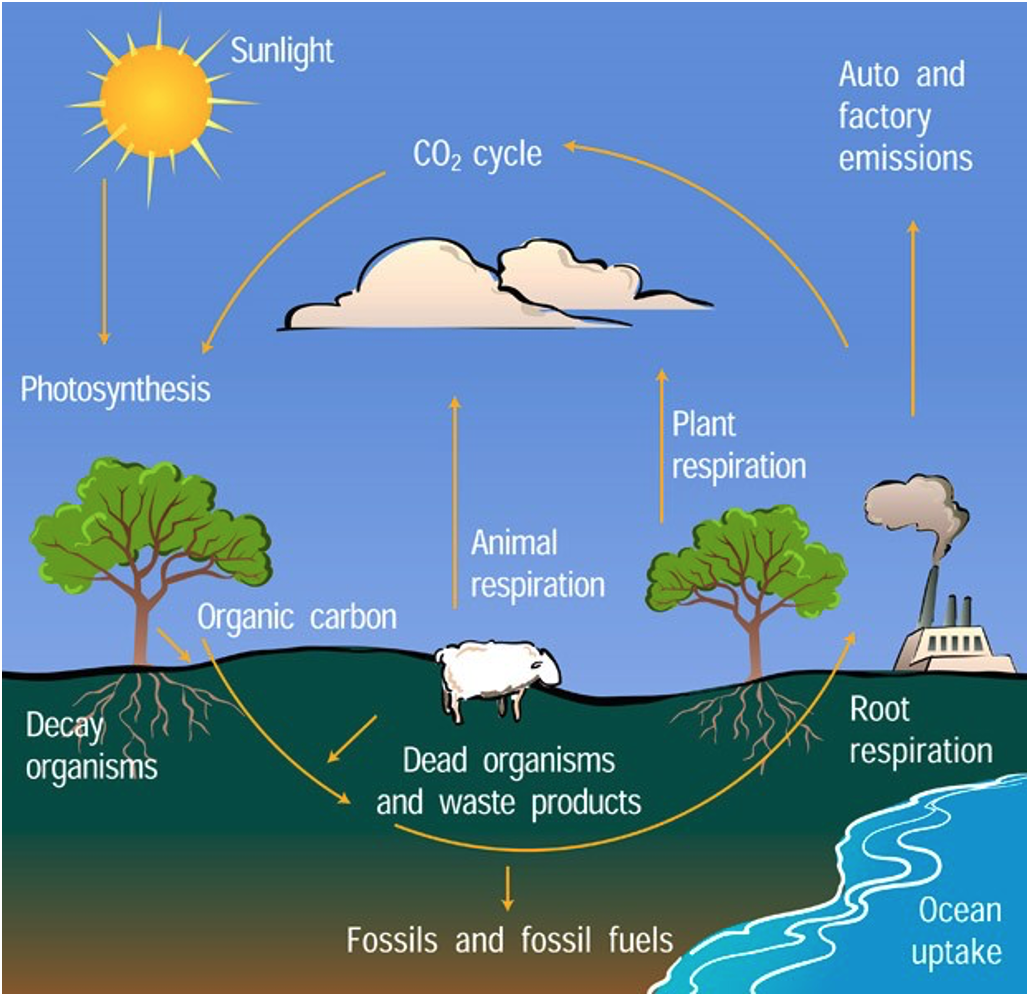
The carbon cycle illustrates the role of soil in the global C cycle. There is more C stored in soil than in the atmosphere and above-ground biomass combined! Soil C is in the form of organic compounds originally created through photosynthesis, in which plants convert atmospheric \(\ce{CO2}\) into plant matter, and enter the soil system as plants and animals die and decompose. Soil organisms consume the organic matter extracting energy and nutrients and releasing water, heat, and \(\ce{CO2}\) back into the atmosphere. If organic matter is added to the soil at a faster rate than organisms convert it to \(\ce{CO2}\), C will gradually be removed from the atmosphere and sequestered in the soil.
Cultivation aerates the soil, triggering increased biological activity, and therefore rapid decomposition, loss of SOM and the release of \(\ce{CO2}\) into the atmosphere. Most soil C losses occur in the first several years after cultivation begins, and currently farmers and scientists are interested in reversing that effect by increasing C stored in the soils through management. (Source: NRCS East National Technology Support Center)
NUTRIENT AVAILABILITY
In addition to nutrient cycling, the actual availability of those nutrients to the plants is critical to consider when assessing soil health. Certain nutrients are only available under specific soil conditions. Soil pH is especially important when it comes to nutrient availability. Consider the following figure:
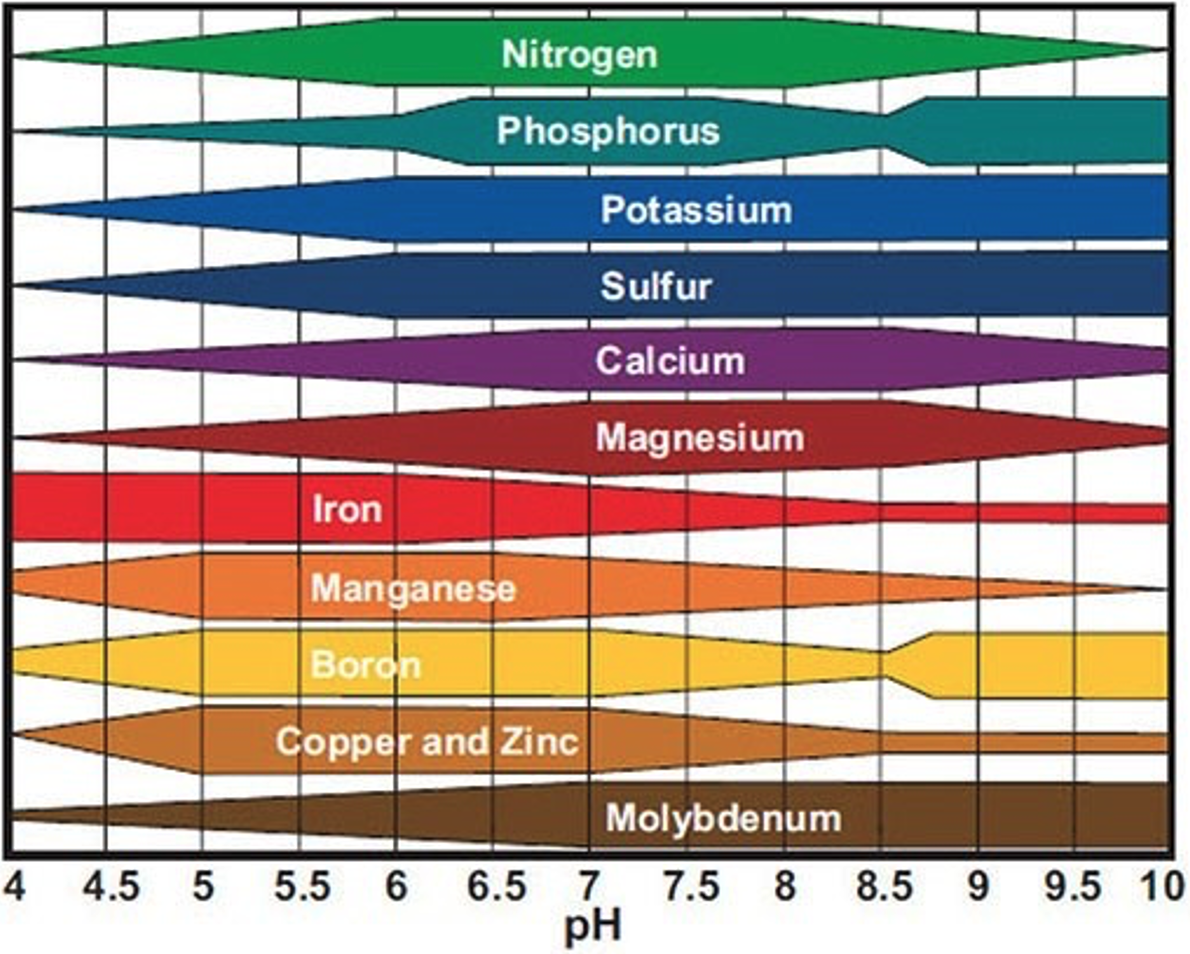
The width of the bar indicates the relative range of availability for each nutrient at various pH levels. Thicker bar widths reflect increased availability, and thinner sections of the bar reflect conditions at which nutrient availability is decreased.
Read through and complete the following activity and questions. All questions should be answered and completed labs are due at the end of the laboratory period. No late work will be accepted.