2.1: Introduction
- Page ID
- 14499
\( \newcommand{\vecs}[1]{\overset { \scriptstyle \rightharpoonup} {\mathbf{#1}} } \)
\( \newcommand{\vecd}[1]{\overset{-\!-\!\rightharpoonup}{\vphantom{a}\smash {#1}}} \)
\( \newcommand{\id}{\mathrm{id}}\) \( \newcommand{\Span}{\mathrm{span}}\)
( \newcommand{\kernel}{\mathrm{null}\,}\) \( \newcommand{\range}{\mathrm{range}\,}\)
\( \newcommand{\RealPart}{\mathrm{Re}}\) \( \newcommand{\ImaginaryPart}{\mathrm{Im}}\)
\( \newcommand{\Argument}{\mathrm{Arg}}\) \( \newcommand{\norm}[1]{\| #1 \|}\)
\( \newcommand{\inner}[2]{\langle #1, #2 \rangle}\)
\( \newcommand{\Span}{\mathrm{span}}\)
\( \newcommand{\id}{\mathrm{id}}\)
\( \newcommand{\Span}{\mathrm{span}}\)
\( \newcommand{\kernel}{\mathrm{null}\,}\)
\( \newcommand{\range}{\mathrm{range}\,}\)
\( \newcommand{\RealPart}{\mathrm{Re}}\)
\( \newcommand{\ImaginaryPart}{\mathrm{Im}}\)
\( \newcommand{\Argument}{\mathrm{Arg}}\)
\( \newcommand{\norm}[1]{\| #1 \|}\)
\( \newcommand{\inner}[2]{\langle #1, #2 \rangle}\)
\( \newcommand{\Span}{\mathrm{span}}\) \( \newcommand{\AA}{\unicode[.8,0]{x212B}}\)
\( \newcommand{\vectorA}[1]{\vec{#1}} % arrow\)
\( \newcommand{\vectorAt}[1]{\vec{\text{#1}}} % arrow\)
\( \newcommand{\vectorB}[1]{\overset { \scriptstyle \rightharpoonup} {\mathbf{#1}} } \)
\( \newcommand{\vectorC}[1]{\textbf{#1}} \)
\( \newcommand{\vectorD}[1]{\overrightarrow{#1}} \)
\( \newcommand{\vectorDt}[1]{\overrightarrow{\text{#1}}} \)
\( \newcommand{\vectE}[1]{\overset{-\!-\!\rightharpoonup}{\vphantom{a}\smash{\mathbf {#1}}}} \)
\( \newcommand{\vecs}[1]{\overset { \scriptstyle \rightharpoonup} {\mathbf{#1}} } \)
\( \newcommand{\vecd}[1]{\overset{-\!-\!\rightharpoonup}{\vphantom{a}\smash {#1}}} \)
\(\newcommand{\avec}{\mathbf a}\) \(\newcommand{\bvec}{\mathbf b}\) \(\newcommand{\cvec}{\mathbf c}\) \(\newcommand{\dvec}{\mathbf d}\) \(\newcommand{\dtil}{\widetilde{\mathbf d}}\) \(\newcommand{\evec}{\mathbf e}\) \(\newcommand{\fvec}{\mathbf f}\) \(\newcommand{\nvec}{\mathbf n}\) \(\newcommand{\pvec}{\mathbf p}\) \(\newcommand{\qvec}{\mathbf q}\) \(\newcommand{\svec}{\mathbf s}\) \(\newcommand{\tvec}{\mathbf t}\) \(\newcommand{\uvec}{\mathbf u}\) \(\newcommand{\vvec}{\mathbf v}\) \(\newcommand{\wvec}{\mathbf w}\) \(\newcommand{\xvec}{\mathbf x}\) \(\newcommand{\yvec}{\mathbf y}\) \(\newcommand{\zvec}{\mathbf z}\) \(\newcommand{\rvec}{\mathbf r}\) \(\newcommand{\mvec}{\mathbf m}\) \(\newcommand{\zerovec}{\mathbf 0}\) \(\newcommand{\onevec}{\mathbf 1}\) \(\newcommand{\real}{\mathbb R}\) \(\newcommand{\twovec}[2]{\left[\begin{array}{r}#1 \\ #2 \end{array}\right]}\) \(\newcommand{\ctwovec}[2]{\left[\begin{array}{c}#1 \\ #2 \end{array}\right]}\) \(\newcommand{\threevec}[3]{\left[\begin{array}{r}#1 \\ #2 \\ #3 \end{array}\right]}\) \(\newcommand{\cthreevec}[3]{\left[\begin{array}{c}#1 \\ #2 \\ #3 \end{array}\right]}\) \(\newcommand{\fourvec}[4]{\left[\begin{array}{r}#1 \\ #2 \\ #3 \\ #4 \end{array}\right]}\) \(\newcommand{\cfourvec}[4]{\left[\begin{array}{c}#1 \\ #2 \\ #3 \\ #4 \end{array}\right]}\) \(\newcommand{\fivevec}[5]{\left[\begin{array}{r}#1 \\ #2 \\ #3 \\ #4 \\ #5 \\ \end{array}\right]}\) \(\newcommand{\cfivevec}[5]{\left[\begin{array}{c}#1 \\ #2 \\ #3 \\ #4 \\ #5 \\ \end{array}\right]}\) \(\newcommand{\mattwo}[4]{\left[\begin{array}{rr}#1 \amp #2 \\ #3 \amp #4 \\ \end{array}\right]}\) \(\newcommand{\laspan}[1]{\text{Span}\{#1\}}\) \(\newcommand{\bcal}{\cal B}\) \(\newcommand{\ccal}{\cal C}\) \(\newcommand{\scal}{\cal S}\) \(\newcommand{\wcal}{\cal W}\) \(\newcommand{\ecal}{\cal E}\) \(\newcommand{\coords}[2]{\left\{#1\right\}_{#2}}\) \(\newcommand{\gray}[1]{\color{gray}{#1}}\) \(\newcommand{\lgray}[1]{\color{lightgray}{#1}}\) \(\newcommand{\rank}{\operatorname{rank}}\) \(\newcommand{\row}{\text{Row}}\) \(\newcommand{\col}{\text{Col}}\) \(\renewcommand{\row}{\text{Row}}\) \(\newcommand{\nul}{\text{Nul}}\) \(\newcommand{\var}{\text{Var}}\) \(\newcommand{\corr}{\text{corr}}\) \(\newcommand{\len}[1]{\left|#1\right|}\) \(\newcommand{\bbar}{\overline{\bvec}}\) \(\newcommand{\bhat}{\widehat{\bvec}}\) \(\newcommand{\bperp}{\bvec^\perp}\) \(\newcommand{\xhat}{\widehat{\xvec}}\) \(\newcommand{\vhat}{\widehat{\vvec}}\) \(\newcommand{\uhat}{\widehat{\uvec}}\) \(\newcommand{\what}{\widehat{\wvec}}\) \(\newcommand{\Sighat}{\widehat{\Sigma}}\) \(\newcommand{\lt}{<}\) \(\newcommand{\gt}{>}\) \(\newcommand{\amp}{&}\) \(\definecolor{fillinmathshade}{gray}{0.9}\)Soils don’t simply exist on the landscape; rather they grow, develop, erode, and slowly transform into other soils through time—a group of processes known collectively as pedogenesis. Pedogenesis begins with parent material, the stuff from which soils form. Although a small minority of soils develop in organic deposits, most derived from inorganic parent materials acted upon by additions, losses, translocations, and transformations
A major type of transformation is weathering of geologic parent materials. All mineral soils develop from the rocks and minerals of the earth's crust. Through weathering, minerals and rocks physically disintegrate into smaller particle sizes, and chemicaly decompose into altered chemical and mineralogical products. However, the original rock material commonly is transported or reworked by some mechanism before a soil is formed. Variations in the history of the initial material lead to a range of types of soil parent materials (Table 1). Additional y, these weathering processes release elements, including plant and animal nutrients, and convert primary minerals into clays.
Mode of transportation | Mode of deposition | Name of parent material |
---|---|---|
Gravity | Gravity | Colluvium |
Water | Stream | Alluvium |
Lake | Lacustrine | |
Ocean | Marine | |
Ice | Ice | Till, moraine |
Meltwater | Outwash (alluvium, lacustrine) | |
Wind | Wind | Loess, dunes |
These parent materials continue to weather over time based on a function of soil forming factors and processes. Another major consideration for weathering potential of soil parent materials is hillslope position. Hillslope position references where on the landscape a soil is located (Figure 1). Hillslope positions essential y delegate the degree of slope and interaction with the groundwater table for the soils of a specific position location. These relationships between soil and slope, or the groundwater table, greatly influence weathering processes over time, increasing both physical and chemical weathering, depending on hil slope position.
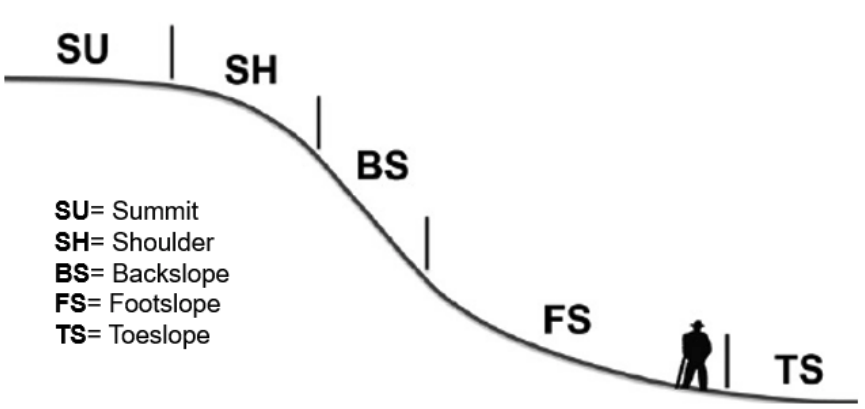
MINERALS
In geology and soil science, a mineral is a naturally occurring, inorganic, crystalline solid having a definite chemical composition and predictable physical properties. Of the six criteria included in this definition, three (naturally occurring, inorganic, and solid) need little elaboration; the remaining three are explained in succeeding paragraphs.
Crystalinity of Minerals
Crystalline means that a mineral’s atoms are arranged in an orderly and repeatable manner; they are not random. The atomic arrangement, which varies among mineral groups, contributes to several of the mineral’s physical properties, including hardness and cleavage, which are explained under “Physical Properties of Minerals.” In contrast, a substance having randomly arranged atoms is amorphous, or noncrystalline, and as such is not considered to be a mineral. A good example of amorphous material is the rock obsidian, a form of volcanic glass.
Chemical Composition of Minerals
Minerals are made up of one or more elements, which give rise to definite chemical compositions (Figure 2). Although chemical formulas are precisely fixed for some minerals (e.g., quartz is SiO2 and orthoclase is KAlSi3O8), they can vary within a narrow range for other minerals (e.g., plagioclase ranges from NaAlSi3O8 to CaAl2Si2O8 because Na+ and Ca2+, which substitute for each other, occur together in varying proportions in the same mineral). Also, no matter how precise the formula, irregularities occur in nature, allowing impurities to sneak in. Note the elements and minerals in Figure 2. What do the four minerals have in common?
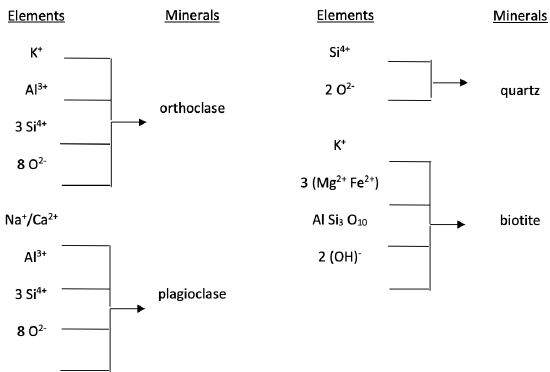
On a mass basis, nearly three-fourths of Earth’s crust is made up of only two elements: oxygen and silicon; and on a volume basis, these two elements constitute more than 90%! The next six most common elements bring the totals to about 98.5 percent by mass, and to nearly 100 percent by volume (Table 2). Obviously, very little room is left for the remaining 100 or so elements, including most plant nutrients, found in the crust. How is it then that soils, nearly all of which derive ultimately from crustal weathering, can supply enough nutrient elements for nearly all terrestrial life, including three-fourths of humanity’s food supply? Your work in this laboratory exercise should help you begin to understand and appreciate the answer to this question.
Element | Ionic Formula | Mass Percent | Volume Percent |
---|---|---|---|
Oxygen |
O2- |
46.60 |
91.97 |
Silicon |
Si4+ |
27.72 | 0.80 |
Aluminum |
Al3+ |
8.13 |
0.77 |
Iron | Fe2+ & Fe3+ | 5.00 | 0.68 |
Calcium | Ca2+ | 3.63 | 1.48 |
Sodium | Na+ | 2.83 | 1.60 |
Potassium | K+ | 2.59 | 2.14 |
Magnesium | Mg2+ | 2.09 | 0.56 |
Mineral Classification Based on Chemical Composition
Most minerals are grouped into seven classes based on their fundamental anionic unit (Table 3). (A few other classes exist, but these can be ignored for this laboratory). Of these, the silicates are by far the most common; nonetheless weathering products of others can be equal y vital.
Mineral Class | Example | Chemical Composition (specimen #) |
Comments |
---|---|---|---|
Sulfides |
1) pyrite 2) sphalerite |
FeS2 (19) ZnS (17) |
readily oxidized in moist soils |
Oxides | hematite | Fe2O3 (15) |
readily oxidized, producing red colors |
Halides |
1) halite 2) sylvite |
NaCl (18) KCl |
rock salt; sometimes found in desert soils |
Carbonates |
1) calcite 2) dolomite |
CaCO3 (13) CaMg(CO3)2 |
1) calcareous; reacts to acid; weathers easily in moist soils 2) calcareous; less reactive than calcite |
Sulfates | gypsum | CaSO4•2H2O (20) |
a common agricultural amendment; sometimes found in desert soils |
Phosphates | apatite | Ca5(PO4)3(F,Cl,OH) (8) |
the only significant source of phosphorus |
Silicates | olivine | (Mg, Fe)2SiO4 |
most easily weathered of the silicates |
pyroxene: augite | (Ca, Na)(Mg, Fe, Al)(Si, Al)2O6 (12) | susceptible to oxidation and hydration | |
amphibole: hornblende |
(Ca,Na)2-3 (Mg,Fe,Al)5Si6(Si,Al)2O22(OH)2 (11) |
susceptible to oxidation and hydration, but more resistant than augite | |
micas: 1) muscovite 2) biotite |
1) KAl2(AlSi3O10)(OH)2 (6) 2) K(Mg, Fe)3(AlSi3O10)(OH)2 (7) |
1) soft, but strongly resists chemical weathering 2) soft; weathers more easily than muscovite |
|
feldspars: 1) orthoclase 2) plagioclase |
1) KAlSi3O8 (9) 2) Na(AlSi3O8)→Ca(Al2Si2O8) (10) |
Most common minerals 1)hard, resistant 2)hard, weathers |
|
quartz | SiO2 (4) |
hard and most resistant to weathering |
Physical Properties of Minerals
Color: Minerals exist in nearly every color, and although color is easy to determine, it might not be reliable for identifying a mineral. For example, pyrite is nearly always brassy yellow, but quartz can range across several hues, values, and chromas from colorless to white, pink, purple, and gray. In some minerals, color can be strongly influenced by impurities.
Luster: independent of a mineral’s color and determined by the nature of light reflected from a mineral’s surface. Most lusters fall into two main groups: metallic and nonmetallic. Metallic lusters are typical of minerals that strongly absorb light, and as a result are opaque, even in very thin pieces. These minerals tend to look like metals, even though their surfaces might range from shiny to dull. Nonmetallic lusters are seen in minerals that allow light to pass through thinly cut slices. Surfaces of nonmetallic minerals might be brilliant like diamond, glassy like quartz, waxy like serpentine, or earthy like clay.
Cleavage and fracture: Cleavage is a mineral’s tendency to break along certain predictable directions when the mineral is struck by a hammer. The resulting cleavage surfaces might be smooth and distinct, or they might be rough and indistinct, depending on the type of mineral. Different minerals also cleave along different directions and at different angles, depending on the type and orientation of atomic bonding. Although the number of possible cleavage directions is 1, 2, 3, 4, or 6, we will focus only on minerals that have 1 (e.g., biotite), 2 (e.g., orthoclase), and 3 (e.g., calcite) directions (Figure 3). Some minerals, when hit by a hammer, do not break along predictable planes: they simply shatter in any direction. These minerals are said to fracture, rather than cleave. Quartz is a good example of a mineral that exhibits fracture.
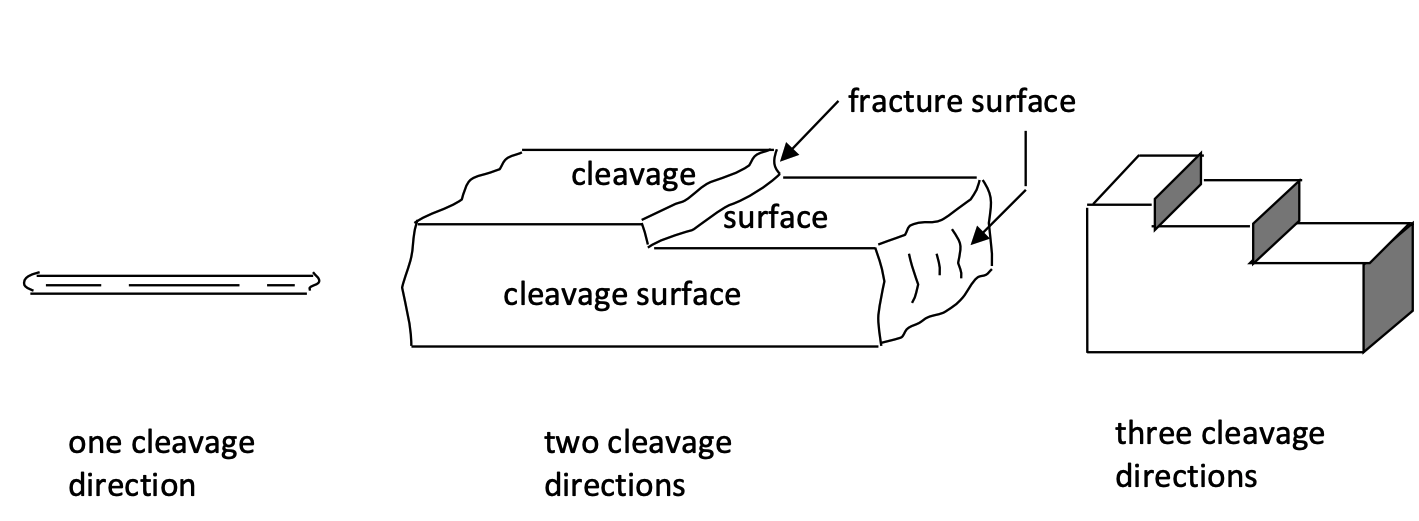
Hardness: Hardness is determined by the resistance of a mineral’s surface to scratching by various instruments of known hardness. It is judged by the Mohs hardness scale, which uses certain reference minerals ranked from 1 (soft) to 10 (hard): (see next page
*Mohs Hardness Scale*
- Talc (easily "scratched" by rubbing between fingers, giving a soapy feel)
- Gypsum (easily scratched by a fingernail)
- Calcite (same hardness as a penny)
- Fluorite
- Apatite (a bit softer than a pocket knife blade)
- Orthoclase (harder than a knife blade, but slightly softer than glass)
- Quartz (harder than both a knife blade and glass)
- Topaz
- Corundum
- Diamond
(Soil minerals are very rarely harder than 7)
Essential elements for plants and animals
Essential elements are those that organisms need to grow and complete their life cycles. For plants, sixteen elements are universal y recognized as essential; nonetheless research conducted in the late 20th century suggests that the number is eighteen or perhaps even greater. We wil consider that plants require eighteen elements (Table 4), although you should understand that many plants, depending on their species, take up more than they seem to need.
A mnemonic phrase can help you memorize the 18 elements:
C HOPKNS CaFe Mg B Mn CuZn Cl CoMo Ni
Translated, this reads “C Hopkns café, managed by “mine” cousins Clyde, Como, and Nicky.”
Note that of the eight most common elements in Earth’s crust, only five, oxygen, iron, calcium, potassium, and magnesium, are among the 18 essential elements (Table 4); and the first of these, oxygen, is supplied by O2 in the atmosphere, not by weathering of minerals and rocks. Of the thirteen remaining essential elements, hydrogen comes from water, carbon comes from carbon dioxide (CO2) in the atmosphere, and nitrogen is maintained by cycling of organic matter. (Nitrogen first is made available to plants by fixation from the atmosphere.) The remaining ten elements, phosphorus, sulfur, boron, manganese, copper, zinc, chlorine, molybdenum, nickel, and cobalt derive from weathering of minerals and rocks
Macronutrients | Micronutrients | ||||
---|---|---|---|---|---|
Element | Atomic Symbol | Plant Available Form | Element | Atomic Symbol | Plant Available Form |
carbon | C | CO2 | iron |
Fe |
Fe2+, Fe3+ |
hydrogen | H | H+ | manganese | Mn | Mn2+ |
oxygen | O | O2, H2O | boron | B | H3BO3 |
nitrogen | M | NH4+, NO3- | zinc | Zn | Zn2+ |
phosphorus | P | H2PO4-, HPO42- | copper | Cu | Cu2+ |
potassium | K | K+ | chlorine | Cl | Cl- |
calcium | Ca | Ca2+ | cobalt | Co | Co2+ |
magnesium | Mg | Mg2+ | molybdenum | Mo | MoO42- |
sulfur | S | SO42- | nickel | Ni | Ni2+ |
In addition to the 18 elements required by plants, animals also require arsenic (As), chromium (Cr), fluorine (F), iodine (I), lithium (Li), sodium (Na), selenium (Se), silicon (Si), and tin (Sn). Herbivorous animals obtain these elements by eating plants, which take up the elements, even though the plant might not need them. Carnivorous animals, in turn, obtain the elements by preying on other animals.
Regardless of whether the elements are required by plants or animals, all except carbon, hydrogen, oxygen, and nitrogen, are made available initially by weathering of minerals. The elements gradually become concentrated in the biosphere through biogeochemical cycles, including the carbon and nitrogen cycles, which will be covered in a later laboratory.
ROCKS
The most common rocks are consolidated assemblages of minerals, but a few (e.g., obsidian, pumice, and coal) comprise non-crystalline (hence, nonmineral) materials. Despite the great number of possible compositions and arrangements, rocks can be divided into three categories based on their mode of formation: igneous, sedimentary, and metamorphic.
Igneous Rocks
Intrusive and Extrusive: Igneous rocks form when molten material called magma cools and solidifies.
Although the magma originates tens of kilometers below Earth’s surface, it can solidify at any depth from its depth of origin to the surface. Magma that remains at depth while cooling and solidifying forms igneous intrusive rocks. Because of extremely slow cooling, minerals can grow to macroscopic size, and can be readily identified in a hand specimen.
Instead of remaining deep throughout the cooling process, some magmas erupt to the surface, where they cool and solidify rapidly. The resulting igneous extrusive rocks can be glassy (i.e., amorphous) or microcrystalline, although many contain a smattering of macrocrystals that formed before eruption. In erupting, the magma might ooze toward the surface as lava, or it might explode violently into the atmosphere, forming pumice and volcanic ash.
Felsic and Mafic: In addition to having distinctively different crystal sizes, igneous rocks also vary in mineralogical composition because of chemical differences among magmas. Some rocks are dominated by quartz and orthoclase (potassium-rich feldspar) because they derived from magma that was rich in silica and potassium. These rocks, which tend to be light colored, are called felsic. Other igneous rocks have little or no quartz or orthoclase, but instead are characterized by calcic plagioclase (calcium-rich feldspar) and ferromagnesian minerals (e.g., augite and hornblende). These are called mafic and are dark colored. Other rocks of intermediate compositions can be found between these extremes.
Classification of Igneous Rocks
The textural trend caused by varying rates of cooling, combined with the mineralogical trend resulting from chemical differences in magma provide the basis for classifying igneous rocks (Table 5). Selected elements also combine to form minerals, which then combine to form a common igneous intrusive rock (Table 6).
Accessory minerals, which can be significant, also vary from felsic to mafic. Of the micas, muscovite is felsic, and biotite is more mafic. As a result, muscovite is found in felsic rocks, including true granite, whereas biotite can occur in rocks ranging from felsic to moderately mafic. An important rock of intermediate composition is granodiorite, which has a characteristic salt-and-pepper appearance derived from light colored quartz and feldspars, especially sodic plagioclase, combined with dark colored biotite. Olivine, a strongly mafic mineral, and augite, which is slightly less mafic, are common constituents of gabbro and basalt.
Table 5. Classification of igneous rocks
Table 6. An example of elements combining to form minerals and minerals combining to form an igneous rock.
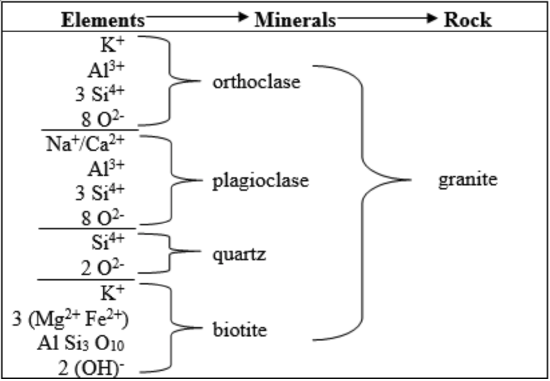
Sedimentary Rocks
Sedimentary rocks, which make up the bulk of Earth’s continental crust, can be either clastic (i.e., fragmental) or nonclastic (i.e., nonfragmental). See Table 7 for examples.
Clastic rock: consist of fragments of previously existing rocks (igneous, sedimentary, or metamorphic) that have been transported to a new location (often an ocean bottom), where they were deposited and buried layer upon layer, and cemented together. The deposits can be several thousand meters thick!
The fragments, or clasts, can vary in size to include clay, silt, sand, gravel, and cobbles.
Nonclastic rock: form by chemical precipitation of dissolved salts and by biochemical precipitation of organically derived compounds in water, most commonly on the floors of shallow seas. Organisms that generate nonclastic rocks include bacteria, algae, diatoms, corals, and mollusks. The most common nonclastic sedimentary rocks are limestone and dolomite (also called dolomitic limestone). Others include rock gypsum, chert, and coal.
Although the two major categories are recognized, most sedimentary rocks are a combination of clastic and nonclastic, nonetheless they usually are dominantly one or the other. For example, the sand grains in sandstone are clastic, but the cement that holds them together can be nonclastic.
Clastic | Nonclastic | ||
---|---|---|---|
Dominant Constituent | Sedimentary Rock | Dominant Constituent | Sedimentary Rock |
clay | claystone | calcite (CaCO3) | limestone |
silt | siltstone | dolomite (CaMg)(CO3)2 | dolomite |
mud (silt + clay) | mudstone | gypsum (CaSO4•2H2O) | gypsum |
thinly layered mud | shale | microcrystalline quartz (SiO2) | chert |
sand (≥ 50%): mostly quartz, feldspars, micas sand: 50-85% sand with remainder mud and possibly some pebbles sand: > 85% sand with remainder mud and possibly pebbles |
sandstones wacke (dirty sandstone) arenite (clean sandstone) |
||
gravel (pebbles) with sand and mud; quartz, orthoclase, quartzite | conglomerate |
Metamorphic Rocks
Metamorphic rocks form primarily by the application of extremely high heat and pressure to some previously existing rock. The original rock may be igneous, sedimentary, or even metamorphic, but sedimentary rocks are most susceptible to metamorphosis. Metamorphic rocks can be either foliated or nonfoliated. Foliated rocks consist of minerals that are aligned or oriented to yield a somewhat stratified or layered appearance, whereas the minerals in nonfoliated rocks are about equally arranged in all directions, giving them a more uniformly massive appearance (Table 8).
Foliated | ||
Metamorphic Rock |
Common Original Rock |
Appearance/Comments |
slate | shale | strongly stratified (layered), flat; often with very fine quartz and mica; cleaves into sheets, but denser than shale |
schist |
shale or other slate |
wavy foliation; often with micas, quartz, feldspars |
gneiss (pronounced “nice”) |
granitic rocks |
minerals oriented to give more faint stratification than schist; often contains the same minerals as its granitic counterpart |
Nonfoliated | ||
marble |
limestone |
calcite cleavage usually visible; fizzes to release CO2 when tested with HCl |
quartzite | quartz arenite | hard; very resistant to weathering |
serpentinite | peridotite (an ultramafic igneous rock) | greenish assemblage of serpentine minerals; high magnesium; occurs intermittently along west coast of North America, and smaller areas of the East Coast |
WEATHERING
Weathering breaks rocks and minerals down, resulting in the production of soils. Weathering occurs via two processes: physical disintegration (breaking into smaller pieces) and chemical decomposition (changing the nature of the minerals themselves).
Physical disintegration causes rock masses to split apart or to abrade and wear away from the larger rock surface. Freezing and thawing, abrasion by particles suspended in wind or water, and grinding caused by glaciers result in disintegration. Repeated actions of these processes cause stresses, which eventually break and erode rock surfaces. Physical weathering usually precedes or occurs in concert with the chemical decomposition process. With a decrease in the size of individual particles, the total number of corners and edges and the total surface area of the rock mass greatly increase.
Chemical decomposition
Chemical decomposition progresses most rapidly when more surface area is available for chemical reactions to occur. Warm and wet environments enhance chemical decomposition. The smaller the particles, the greater the surface area of a given volume of rock (the greater the total exposed corners and edges) and the greater the rate of chemical decomposition. The total number of corners exposed to weathering processes is the major factor in the determination of weathering rates. This is because a corner has three surfaces and three edges for chemical attack. Chemical weathering is a combination of five major processes: carbonation, hydration, hydrolysis, oxidation-reduction (redox), and solution.
Hydrolysis and particle size: Hydrolysis is a chemical decomposition process involving the splitting of water molecules during a reaction. A hydrolysis reaction can be easily identified by looking for water on the left side of a chemical equation and the absence of water (but the formation of H+ and/or OH-) on the right side of the equation. All minerals weather by hydrolysis. The hydrolysis reaction is particularly evident with silicate minerals (e. g. feldspars and micas). Hydrolysis is the primary reaction resulting in the release of most plant nutrient elements from minerals. The mineral orthoclase will weather by hydrolysis.
\[ \mathrm{KAlSi_3O_8 + H_2O \rightarrow HAlSi_3O8 + K^+ + OH^-} \nonumber \]
Orthoclase + Water \(\rightarrow\) Al-silicate mineral + Potassium ion + Hydroxide ion
The potassium cation (K+) released by this hydrolysis reaction is soluble in water and can be adsorbed by the soil clay minerals and organic colloids, used by plants, or be removed (leached) by the drainage water. The aluminum silicate compound may crystallize into a clay mineral (e. g. kaolinite). This natural chemical weathering process occurs at a very slow but continual rate in all rocks, parent materials, and soils. The smaller the original mineral, the faster the hydrolysis reaction will occur. The hydroxide ion (OH-) will increase the pH. When a glacier grinds rocks with glacial ice (water), the grinding is a physical disintegration process. However, the resulting hydrolysis reaction of the fine particles hydrolyzing with the water causes the glacial till to have a high pH (abrasion pH). Initially, the pH of the glacial till will be 9 or 10, but as the glacial till absorbs carbon dioxide from the air, the pH will drop.
Carbonation and Solution: The metabolic activities of plant roots and microorganisms produce an abundance of CO2 within soil pores. This CO2 reacts with soil water to produce most of the carbonic acid found in soils.
\[ CO_2 + H_2O \leftrightarrow H_2CO_3 \nonumber \]
Carbon Dioxide + Water \( \leftrightarrow \) Carbonic acid
The carbonic acid in the soil water continually reacts with minerals as the water percolates through soils and rocks. Carbonic acid, a weak acid, is the most common acid found in soils. Carbonic acid can ionize into acidic hydrogen ions (H+) and bicarbonate (HCO3-):
\[H_2CO_3 \leftrightarrow H^+ + HCO_3 \nonumber \]
Carbonic acid \( \leftrightarrow \) Acidic hydrogen ion + Bicarbonate ion
At pH < 6, this reaction tends to go more to the left than to the right, indicating carbonic acid is a weak acid and does not produce very many acidic hydrogen ions. Carbonic acid is effective in accelerating the chemical decomposition processes of minerals because nature continually provides rainwater and carbon dioxide. Thus, rainwater drives this reaction to the right. As the rainwater moves through the soil, it carries soluble bicarbonate ions deeper into the soil profile and deposits the acidic hydrogen ions on the soil surfaces. The law of mass action allows the soil to eventually become acidic with time of weathering as rainfall drives the above reaction to the right.
As a result of a glacier grinding rocks, the hydrolysis reaction results in the glacial till containing soluble Ca2+ ions and OH- ions (which causes a high pH, greater than 8.3). As the glacial till is exposed to carbonic acid, the glacial till undergoes two carbonation reactions. The first reaction process is quite rapid, while the second reaction is slow and requires time for completion. The first carbonation reaction is
\[ Ca^{2+} + 2OH^- + H_2CO^3 \rightarrow CaCO_3 + 2H_2O \nonumber \]
Soluble calcium + Soluble hydroxide + Carbonic acid \( \rightarrow \) Low soluble calcite + Water
The second reaction is:
\[ CaCO_3 + H_2CO_3 \rightarrow Ca(HCO_3)_2 \nonumber \]
Calcite + Carbonic acid \( \rightarrow \) Soluble calcium bicarbonate
The calcium bicarbonate dissolves slowly and releases soluble calcium ions (Ca2+) for plant uptake or possible leaching downward through the soil when excessive rainfall or irrigation occurs. The loss of the calcite minerals (the chief mineral in limestone) eventually leads to the development of acidic soils, carbonate hardpans, and the formation of huge underground caverns (e. g. Mammoth Cave and Carlsbad Caverns). The cave one walks through is the hole remaining after the soluble calcium bicarbonate has left the cavity where the carbonic acid dissolved the limestone rock.
The soluble calcium and bicarbonate move downward and enter the ground water, which eventually emerges as water in streams flowing to the ocean. The presence of the calcium bicarbonate in the ground water is the major reason limestone ground water is termed “hard water”.
Eventually, the second reaction has two major effects in a high rainfall environment. First, the surface soil will become acidic and the pH will decrease due to increased rainfall and CO2 production (H2CO3). Second, soluble calcium is made available to plants. However, further rainfall will leach the soluble calcium bicarbonate downward.
Eventually, the soil will become depleted of calcium (Ca2+). The same phenomenon will be true for magnesium (Mg2+), potassium (K+), and sodium (Na+) cations released to the soil by the hydrolysis of various minerals. This process results in a loss of overall plant nutrition and soil fertility.
Reduction-oxidation (redox) reactions: involve the transfer of electrons from one reactant to another. In every redox reaction, some substance becomes oxidized and some other substance becomes reduced by the transfer of the electron. The substance losing the electron is oxidized and the component accepting the electrons becomes reduced. Commonly, but not always, oxygen serves as the oxidizing agent.
Oxygen combines with an element in a mineral, such as iron (Fe2+), causing the iron to give up some of its electrons, which are accepted by oxygen. The iron becomes oxidized while the oxygen becomes reduced. These electron transfers constitute a form of chemical weathering, which disrupts the mineral’s atomic structure, releasing several elements, and leading to the development of new compounds including clay minerals.
Although redox reactions take place continuously in soils, their reaction rates can be very slow and unnoticeable. If a soil is moist and well aerated, iron tends to become oxidized (Fe3+, loses electrons to oxygen), but if air (oxygen gas) is absent, as occurs with water-saturated soil, the iron becomes reduced (Fe2+, gains electrons). The extent of either oxidation or reduction in soils is manifested in the color of the soil. Oxidized iron (Fe3+) occurs where oxygen is present in well-drained soils and produces red, yellow, or brown colors in soils. Reduced iron (Fe2+) exists in flooded or very poorly drained soils and produces black, gray, olive, and blue colors. Alternating periods of oxidation and reduction, caused by fluctuating water levels in a soil, result in mottling (blotches of red and yellow mixed with gray colors) to occur. Continuous strongly reducing conditions caused by long-term water-logging forms gleyed conditions. Gleyed soils have dark gray to blue to black soil colors.
\[ Fe^{2+} \xleftrightarrow{O_2} Fe^{3+} \nonumber \]
Reduced (gray soil colors) ↔ Oxidized (red and yellow soil colors)
Read through and complete the folowing activity and questions. All questions should be answered and completed labs are due at the end of the laboratory period. No late work wil be accepted.