6.3: Controls over wind direction and speed
- Page ID
- 15187
\( \newcommand{\vecs}[1]{\overset { \scriptstyle \rightharpoonup} {\mathbf{#1}} } \)
\( \newcommand{\vecd}[1]{\overset{-\!-\!\rightharpoonup}{\vphantom{a}\smash {#1}}} \)
\( \newcommand{\id}{\mathrm{id}}\) \( \newcommand{\Span}{\mathrm{span}}\)
( \newcommand{\kernel}{\mathrm{null}\,}\) \( \newcommand{\range}{\mathrm{range}\,}\)
\( \newcommand{\RealPart}{\mathrm{Re}}\) \( \newcommand{\ImaginaryPart}{\mathrm{Im}}\)
\( \newcommand{\Argument}{\mathrm{Arg}}\) \( \newcommand{\norm}[1]{\| #1 \|}\)
\( \newcommand{\inner}[2]{\langle #1, #2 \rangle}\)
\( \newcommand{\Span}{\mathrm{span}}\)
\( \newcommand{\id}{\mathrm{id}}\)
\( \newcommand{\Span}{\mathrm{span}}\)
\( \newcommand{\kernel}{\mathrm{null}\,}\)
\( \newcommand{\range}{\mathrm{range}\,}\)
\( \newcommand{\RealPart}{\mathrm{Re}}\)
\( \newcommand{\ImaginaryPart}{\mathrm{Im}}\)
\( \newcommand{\Argument}{\mathrm{Arg}}\)
\( \newcommand{\norm}[1]{\| #1 \|}\)
\( \newcommand{\inner}[2]{\langle #1, #2 \rangle}\)
\( \newcommand{\Span}{\mathrm{span}}\) \( \newcommand{\AA}{\unicode[.8,0]{x212B}}\)
\( \newcommand{\vectorA}[1]{\vec{#1}} % arrow\)
\( \newcommand{\vectorAt}[1]{\vec{\text{#1}}} % arrow\)
\( \newcommand{\vectorB}[1]{\overset { \scriptstyle \rightharpoonup} {\mathbf{#1}} } \)
\( \newcommand{\vectorC}[1]{\textbf{#1}} \)
\( \newcommand{\vectorD}[1]{\overrightarrow{#1}} \)
\( \newcommand{\vectorDt}[1]{\overrightarrow{\text{#1}}} \)
\( \newcommand{\vectE}[1]{\overset{-\!-\!\rightharpoonup}{\vphantom{a}\smash{\mathbf {#1}}}} \)
\( \newcommand{\vecs}[1]{\overset { \scriptstyle \rightharpoonup} {\mathbf{#1}} } \)
\( \newcommand{\vecd}[1]{\overset{-\!-\!\rightharpoonup}{\vphantom{a}\smash {#1}}} \)
\(\newcommand{\avec}{\mathbf a}\) \(\newcommand{\bvec}{\mathbf b}\) \(\newcommand{\cvec}{\mathbf c}\) \(\newcommand{\dvec}{\mathbf d}\) \(\newcommand{\dtil}{\widetilde{\mathbf d}}\) \(\newcommand{\evec}{\mathbf e}\) \(\newcommand{\fvec}{\mathbf f}\) \(\newcommand{\nvec}{\mathbf n}\) \(\newcommand{\pvec}{\mathbf p}\) \(\newcommand{\qvec}{\mathbf q}\) \(\newcommand{\svec}{\mathbf s}\) \(\newcommand{\tvec}{\mathbf t}\) \(\newcommand{\uvec}{\mathbf u}\) \(\newcommand{\vvec}{\mathbf v}\) \(\newcommand{\wvec}{\mathbf w}\) \(\newcommand{\xvec}{\mathbf x}\) \(\newcommand{\yvec}{\mathbf y}\) \(\newcommand{\zvec}{\mathbf z}\) \(\newcommand{\rvec}{\mathbf r}\) \(\newcommand{\mvec}{\mathbf m}\) \(\newcommand{\zerovec}{\mathbf 0}\) \(\newcommand{\onevec}{\mathbf 1}\) \(\newcommand{\real}{\mathbb R}\) \(\newcommand{\twovec}[2]{\left[\begin{array}{r}#1 \\ #2 \end{array}\right]}\) \(\newcommand{\ctwovec}[2]{\left[\begin{array}{c}#1 \\ #2 \end{array}\right]}\) \(\newcommand{\threevec}[3]{\left[\begin{array}{r}#1 \\ #2 \\ #3 \end{array}\right]}\) \(\newcommand{\cthreevec}[3]{\left[\begin{array}{c}#1 \\ #2 \\ #3 \end{array}\right]}\) \(\newcommand{\fourvec}[4]{\left[\begin{array}{r}#1 \\ #2 \\ #3 \\ #4 \end{array}\right]}\) \(\newcommand{\cfourvec}[4]{\left[\begin{array}{c}#1 \\ #2 \\ #3 \\ #4 \end{array}\right]}\) \(\newcommand{\fivevec}[5]{\left[\begin{array}{r}#1 \\ #2 \\ #3 \\ #4 \\ #5 \\ \end{array}\right]}\) \(\newcommand{\cfivevec}[5]{\left[\begin{array}{c}#1 \\ #2 \\ #3 \\ #4 \\ #5 \\ \end{array}\right]}\) \(\newcommand{\mattwo}[4]{\left[\begin{array}{rr}#1 \amp #2 \\ #3 \amp #4 \\ \end{array}\right]}\) \(\newcommand{\laspan}[1]{\text{Span}\{#1\}}\) \(\newcommand{\bcal}{\cal B}\) \(\newcommand{\ccal}{\cal C}\) \(\newcommand{\scal}{\cal S}\) \(\newcommand{\wcal}{\cal W}\) \(\newcommand{\ecal}{\cal E}\) \(\newcommand{\coords}[2]{\left\{#1\right\}_{#2}}\) \(\newcommand{\gray}[1]{\color{gray}{#1}}\) \(\newcommand{\lgray}[1]{\color{lightgray}{#1}}\) \(\newcommand{\rank}{\operatorname{rank}}\) \(\newcommand{\row}{\text{Row}}\) \(\newcommand{\col}{\text{Col}}\) \(\renewcommand{\row}{\text{Row}}\) \(\newcommand{\nul}{\text{Nul}}\) \(\newcommand{\var}{\text{Var}}\) \(\newcommand{\corr}{\text{corr}}\) \(\newcommand{\len}[1]{\left|#1\right|}\) \(\newcommand{\bbar}{\overline{\bvec}}\) \(\newcommand{\bhat}{\widehat{\bvec}}\) \(\newcommand{\bperp}{\bvec^\perp}\) \(\newcommand{\xhat}{\widehat{\xvec}}\) \(\newcommand{\vhat}{\widehat{\vvec}}\) \(\newcommand{\uhat}{\widehat{\uvec}}\) \(\newcommand{\what}{\widehat{\wvec}}\) \(\newcommand{\Sighat}{\widehat{\Sigma}}\) \(\newcommand{\lt}{<}\) \(\newcommand{\gt}{>}\) \(\newcommand{\amp}{&}\) \(\definecolor{fillinmathshade}{gray}{0.9}\)Air is constantly moving to seek an equilibrium between areas of more air molecules (higher pressure) and those with less (lower pressure). You have probably experienced this by opening a container that has been vacuum packed. Because the container is vacuum packed, there is less air inside the can (lower pressure) than outside the can (higher pressure). When you open the container you here a "whoosh" as air rushes into it. The air rushing from outside the container into it is a wind, albeit at the microscale. Wind is nothing more than the movement of air molecules from one place to the next. The direction and speed of the wind represents the balance between three basic forces acting on it: the pressure gradient, the Coriolis force, and surface friction.
Pressure Gradient
The spacing of isobars indicates the change in pressure over distance otherwise known as a pressure gradient. We can induce a change in pressure over distance by the unequal heating of the Earth's surface. This can be done when one location receives more incoming energy than another, possibly because one place has a higher sun angle than another. Heating the air in one place causes it to rise off the surface promoting low pressure with the pressure increasing away from that location. The creation of a pressure gradient initially causes the air to flow from higher toward lower pressure creating a wind. So in terms of a cause-and-effect relationship:
Energy gradient -> temperature gradient -> pressure gradient -> wind
The orientation or direction of a pressure gradient is always described as being from higher toward lower pressure. The speed of the wind is controlled by the strength of the pressure gradient, the stronger the pressure gradient the higher the wind speed. The strength of the pressure gradient can be discerned from the spacing of isobars on a weather map. Figure PC.4 shows two different pressure gradients. The distance between points A and B and C and D are the same but the amount of pressure change is quite different. You can tell that the pressure gradient is greater between A and B because the spacing of the isobars is much closer than between C and D. As a result, the wind blows much faster between A and B.
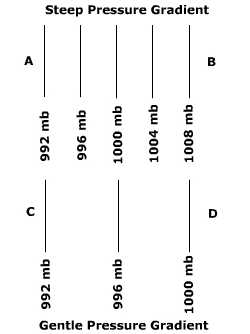
Coriolis Force
The Coriolis force is the effect of earth rotation on the direction of the wind. The Coriolis force arises for two reasons, first our directional system of latitude and longitude has been fixed to a rotating earth. Thus, our frame of reference for monitoring the direction of a free-moving object above the Earth is constantly changing. The second reason is the amount of turning about a vertical axis varies from a maximum at the poles and minimum at the equator. Demonstrate this by standing a pencil on end at the north pole and turn the globe. The pencil completes one full rotation. But standing the pencil on end at the equator and rotating the earth yields no rotation about a vertical axis. Figure \(\PageIndex{1}\) shows the deflection that a missile experiences when shot from the North Pole towards the Equator. Because the Earth, and the target, has rotated underneath the free-moving missile, it appears that the missile has veered off course (changed direction). Such is the case for winds blowing above the surface. The deflection works the same way for an east-west wind, the path will curve to the right as it moves across the surface. Go to Coriolis Force animation
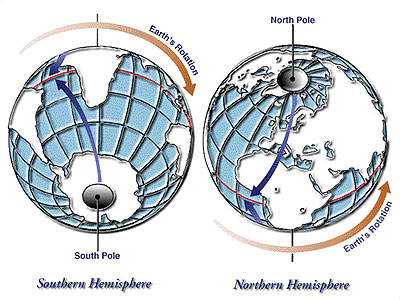
Though the air is deflected to the right of its path in the Northern hemisphere, in the Southern Hemisphere wind is deflected to the left of its path. Why the difference? It all has to do with perspective. Pick up a globe and spin it in a west to east direction. Now look down on it from above the North Pole. It appears to by spinning in counterclockwise direction. Now keep it spinning from west to east, lift the globe over your head and look at it from above the South Pole. It appears to be going in a clockwise direction. (Kind of weird, huh?)
Friction
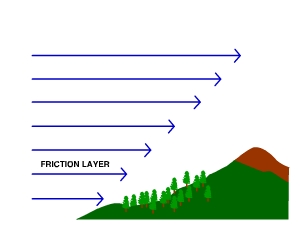
Generally speaking wind speed increases with height above the surface as the frictional force of surface diminishes with height. The friction imposed on air mechanically slows the wind and diverts its direction. The friction layer is the layer of air that is influenced by friction caused by the surface. The friction layer varies in height across the Earth, but for the most part lies within about a kilometer of the surface.
Investigate how geoscientist measure and analyze wind by "Digging Deeper: Measuring, Visualizing, and Analyzing Wind Direction and Speed" or skip and continue reading.
There are two basic measurements taken of wind conditions, speed and direction. As noted in this chapter, wind speed is simply how fast the wind is blowing. Wind direction is described as where the wind comes from, not where it's going to. Let's dig deeper into how scientists measure and visualize wind speed and direction when analyzing weather.
Instrumentation
Wind speed is measured using an anemometer. A typical anemometer consists of 3 or 4 spinning "cups" mounted at equal angles to one another and then attached to a rod. The cups rotate proportional to the air flow past them. The number of revolutions in a unit of time is converted into a wind speed and recorded on a data logging device or read from dial. The speed is reported in miles per hour, kilometers per hour, or knots.
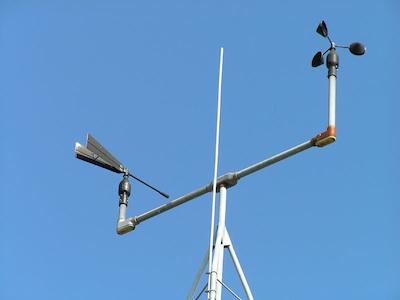
The Beaufort Scale was created in 1805 by Sir Francis Beaufort as a qualitative measure of wind condition effects on the sails of his ship. Thirteen classes, from 0 to 12, determined the number of sails to hoist. At 0 all sails would be raised with just enough wind to give steerage. At 6 half his sails would be up, and at 12 all sails would be down as conditions were to severe for canvas sails to withstand them. In 1906, the descriptions were changed to how seas behaved under different wind conditions. The Beaufort scale continues to be used by citizen weather spotters to estimate of conditions in the absence of instrumentation during severe weather.
Table \(\PageIndex{1}\) Beaufort Scale (Courtesy NOAA: Source)
A wind vane is a instrument to measure wind direction (on left in Table \(\PageIndex{1}\) above). At its most simple configuration it is a pointed rod with a vane mounted perpendicular to the rod and attached to a vertical pole on which it rotates. The vane acts to orient the pointed end into the wind and hence points to the direction the wind comes from.
An aerovane is an instrument that can measure both wind direction and speed simultaneously. The aerovane sits atop a pole and rotates as the wind direction changes. The propeller spins faster as the wind speed increases. The spin of the propeller at the front spins faster as the wind speed increases. A signal is sent to a data logger where the orientation of the aerovane and spin of the propeller is converted into units of wind direction and speed. A vane on the tail orients the instrument into the wind.
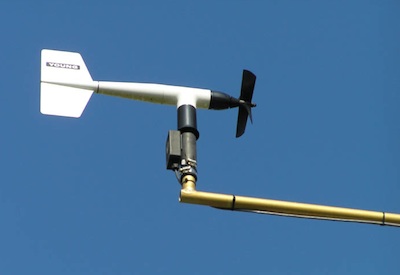
Visualization and Analysis
Once wind data has been recorded it can be archived in print, or more likely digital form and symbolized on maps for further analysis.
Wind direction
Wind direction can be reported as a direction or in degrees. Wind direction is symbolized on a weather map with a line radiating out from a point in the direction of the wind. The direction of the wind as symbolized in figure \(\PageIndex{6}\) is northeast.
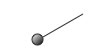
The prevailing wind the the direction that the wind comes from the most often. It can be determined by measuring the wind direction at many different times for a particular location and the calculating the percentage of times the wind comes from different directions. A wind rose is used to visualize the prevailing wind wind. The "rose" is constructed with bars or lines placed around a circle and drawn to a length proportional to the amount of time the wind blows from different directions. The prevailing wind shown in figure 6-D is from the south. Wind barbsor a color code can be added to show the average speed from a given direction.
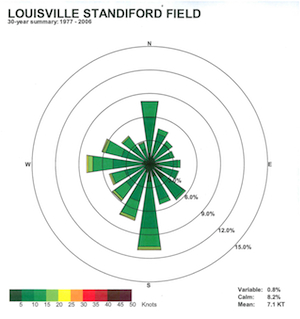
Wind speed
Wind speed is recorded in either miles per hour, kilometers per hour or knots. Small barbs or triangles attached to weather direction symbols are used to visualize wind speed on weather maps. Given that wind speed fluctuates so much over a short period of time, the symbols represent ranges of wind speed.
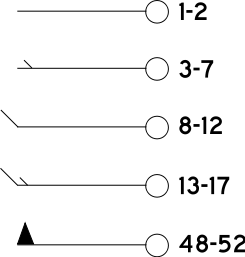
A "rule of thumb" for remembering the symbols is, half barb=5 knots, full barb=10 knots, flag=50 knots.
The patterns of wind speed and direction can be shown on weather maps, like the one in Figure 6-F. As described earlier in the chapter, the pressure gradient determines the speed of the wind. Note how the wind speed is greater where the isobars are closer together. The wind direction symbols show the typical clockwise movement around highs, and counterclockwise around lows. Local conditions at the time the data was collected may show some deviation from this generalization.
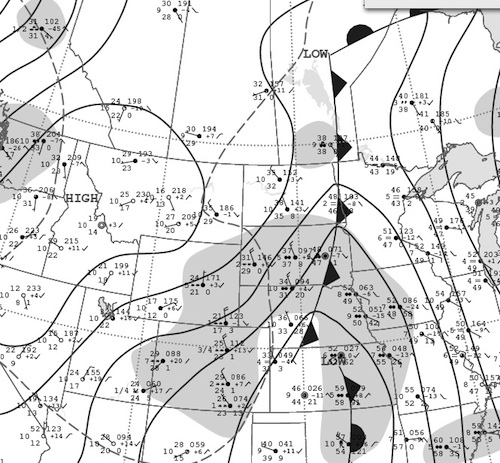
Surface circulation around highs and lows.
The combined effects of the pressure gradient, Coriolis effect, surface friction is shown in Figure \(\PageIndex{10}\). Recall that air always flows from higher towards lower pressure. Around high pressure systems (H), air is directed outward from the center. Around low pressure systems (L) air is directed inward toward the center. If pressure gradient was the only force acting on the air, wind would move directly across isobars at a perpedicular angle. Wind instead moves across the isobars at an angle of anywhere from 10o to 45o. Because the Coriolis Effect bends the air to the right of of it's path (i.e. the direction of the pressure gradient) in the Northern hemisphere, air takes on a clockwise flow around highs and counterclockwise around lows. In the Southern hemisphere, air circulates in a counterclockwise fashion around highs and clockwise around lows.
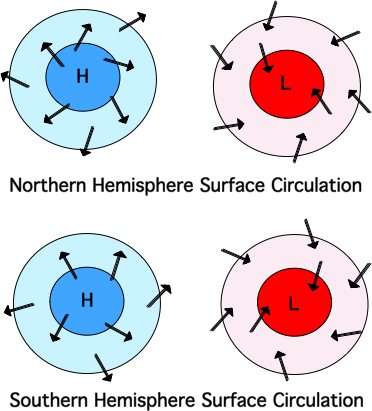
Above the "friction layer", only the pressure gradient and Coriolis effect operate on wind. At particular latitudes, the opposing pressure gradient and Coriolis forces can balance one another high in the troposphere above the friction layer. When this occurs, winds tend to blow parallel to isobars. Winds that blow roughly parallel to isobars are called "geostrophic winds". The fast-moving jet streams are type of geostrophic wind.