12.2: Currents and Tides
- Page ID
- 32242
\( \newcommand{\vecs}[1]{\overset { \scriptstyle \rightharpoonup} {\mathbf{#1}} } \)
\( \newcommand{\vecd}[1]{\overset{-\!-\!\rightharpoonup}{\vphantom{a}\smash {#1}}} \)
\( \newcommand{\id}{\mathrm{id}}\) \( \newcommand{\Span}{\mathrm{span}}\)
( \newcommand{\kernel}{\mathrm{null}\,}\) \( \newcommand{\range}{\mathrm{range}\,}\)
\( \newcommand{\RealPart}{\mathrm{Re}}\) \( \newcommand{\ImaginaryPart}{\mathrm{Im}}\)
\( \newcommand{\Argument}{\mathrm{Arg}}\) \( \newcommand{\norm}[1]{\| #1 \|}\)
\( \newcommand{\inner}[2]{\langle #1, #2 \rangle}\)
\( \newcommand{\Span}{\mathrm{span}}\)
\( \newcommand{\id}{\mathrm{id}}\)
\( \newcommand{\Span}{\mathrm{span}}\)
\( \newcommand{\kernel}{\mathrm{null}\,}\)
\( \newcommand{\range}{\mathrm{range}\,}\)
\( \newcommand{\RealPart}{\mathrm{Re}}\)
\( \newcommand{\ImaginaryPart}{\mathrm{Im}}\)
\( \newcommand{\Argument}{\mathrm{Arg}}\)
\( \newcommand{\norm}[1]{\| #1 \|}\)
\( \newcommand{\inner}[2]{\langle #1, #2 \rangle}\)
\( \newcommand{\Span}{\mathrm{span}}\) \( \newcommand{\AA}{\unicode[.8,0]{x212B}}\)
\( \newcommand{\vectorA}[1]{\vec{#1}} % arrow\)
\( \newcommand{\vectorAt}[1]{\vec{\text{#1}}} % arrow\)
\( \newcommand{\vectorB}[1]{\overset { \scriptstyle \rightharpoonup} {\mathbf{#1}} } \)
\( \newcommand{\vectorC}[1]{\textbf{#1}} \)
\( \newcommand{\vectorD}[1]{\overrightarrow{#1}} \)
\( \newcommand{\vectorDt}[1]{\overrightarrow{\text{#1}}} \)
\( \newcommand{\vectE}[1]{\overset{-\!-\!\rightharpoonup}{\vphantom{a}\smash{\mathbf {#1}}}} \)
\( \newcommand{\vecs}[1]{\overset { \scriptstyle \rightharpoonup} {\mathbf{#1}} } \)
\( \newcommand{\vecd}[1]{\overset{-\!-\!\rightharpoonup}{\vphantom{a}\smash {#1}}} \)
\(\newcommand{\avec}{\mathbf a}\) \(\newcommand{\bvec}{\mathbf b}\) \(\newcommand{\cvec}{\mathbf c}\) \(\newcommand{\dvec}{\mathbf d}\) \(\newcommand{\dtil}{\widetilde{\mathbf d}}\) \(\newcommand{\evec}{\mathbf e}\) \(\newcommand{\fvec}{\mathbf f}\) \(\newcommand{\nvec}{\mathbf n}\) \(\newcommand{\pvec}{\mathbf p}\) \(\newcommand{\qvec}{\mathbf q}\) \(\newcommand{\svec}{\mathbf s}\) \(\newcommand{\tvec}{\mathbf t}\) \(\newcommand{\uvec}{\mathbf u}\) \(\newcommand{\vvec}{\mathbf v}\) \(\newcommand{\wvec}{\mathbf w}\) \(\newcommand{\xvec}{\mathbf x}\) \(\newcommand{\yvec}{\mathbf y}\) \(\newcommand{\zvec}{\mathbf z}\) \(\newcommand{\rvec}{\mathbf r}\) \(\newcommand{\mvec}{\mathbf m}\) \(\newcommand{\zerovec}{\mathbf 0}\) \(\newcommand{\onevec}{\mathbf 1}\) \(\newcommand{\real}{\mathbb R}\) \(\newcommand{\twovec}[2]{\left[\begin{array}{r}#1 \\ #2 \end{array}\right]}\) \(\newcommand{\ctwovec}[2]{\left[\begin{array}{c}#1 \\ #2 \end{array}\right]}\) \(\newcommand{\threevec}[3]{\left[\begin{array}{r}#1 \\ #2 \\ #3 \end{array}\right]}\) \(\newcommand{\cthreevec}[3]{\left[\begin{array}{c}#1 \\ #2 \\ #3 \end{array}\right]}\) \(\newcommand{\fourvec}[4]{\left[\begin{array}{r}#1 \\ #2 \\ #3 \\ #4 \end{array}\right]}\) \(\newcommand{\cfourvec}[4]{\left[\begin{array}{c}#1 \\ #2 \\ #3 \\ #4 \end{array}\right]}\) \(\newcommand{\fivevec}[5]{\left[\begin{array}{r}#1 \\ #2 \\ #3 \\ #4 \\ #5 \\ \end{array}\right]}\) \(\newcommand{\cfivevec}[5]{\left[\begin{array}{c}#1 \\ #2 \\ #3 \\ #4 \\ #5 \\ \end{array}\right]}\) \(\newcommand{\mattwo}[4]{\left[\begin{array}{rr}#1 \amp #2 \\ #3 \amp #4 \\ \end{array}\right]}\) \(\newcommand{\laspan}[1]{\text{Span}\{#1\}}\) \(\newcommand{\bcal}{\cal B}\) \(\newcommand{\ccal}{\cal C}\) \(\newcommand{\scal}{\cal S}\) \(\newcommand{\wcal}{\cal W}\) \(\newcommand{\ecal}{\cal E}\) \(\newcommand{\coords}[2]{\left\{#1\right\}_{#2}}\) \(\newcommand{\gray}[1]{\color{gray}{#1}}\) \(\newcommand{\lgray}[1]{\color{lightgray}{#1}}\) \(\newcommand{\rank}{\operatorname{rank}}\) \(\newcommand{\row}{\text{Row}}\) \(\newcommand{\col}{\text{Col}}\) \(\renewcommand{\row}{\text{Row}}\) \(\newcommand{\nul}{\text{Nul}}\) \(\newcommand{\var}{\text{Var}}\) \(\newcommand{\corr}{\text{corr}}\) \(\newcommand{\len}[1]{\left|#1\right|}\) \(\newcommand{\bbar}{\overline{\bvec}}\) \(\newcommand{\bhat}{\widehat{\bvec}}\) \(\newcommand{\bperp}{\bvec^\perp}\) \(\newcommand{\xhat}{\widehat{\xvec}}\) \(\newcommand{\vhat}{\widehat{\vvec}}\) \(\newcommand{\uhat}{\widehat{\uvec}}\) \(\newcommand{\what}{\widehat{\wvec}}\) \(\newcommand{\Sighat}{\widehat{\Sigma}}\) \(\newcommand{\lt}{<}\) \(\newcommand{\gt}{>}\) \(\newcommand{\amp}{&}\) \(\definecolor{fillinmathshade}{gray}{0.9}\)Ocean water moves as waves, currents, and tides. Ocean currents are driven by persistent global winds blowing over the water’s surface and by water density. Ocean currents are part of Earth’s heat engine in which solar energy is absorbed by ocean water and distributed by ocean currents. Water has another unique property, high specific heat, that relates to ocean currents. Specific heat is the amount of heat necessary to raise a unit volume of a substance one degree. For water it takes one calorie per cubic centimeter to raise its temperature one degree Celsius. This means the oceans, covering 71% of the Earth’s surface, soak up solar heat with little temperature change and distribute that heat around the Earth by ocean currents.
Surface Currents
The Earth’s rotation and the Coriolis effect exert significant influence on ocean currents. In the figure, the black arrows show global surface currents. Notice the large circular currents in the Northern and Southern Hemispheres in the Atlantic, Pacific, and Indian Oceans. These are driven by prevailing atmospheric circulation (air movement) and are called gyres [9]. Gyres rotate clockwise in the Northern Hemisphere and counterclockwise in the Southern Hemisphere because of the Coriolis effect, which, due to the Earth's rotation, causes wind and ocean currents to be deflected to the right in the Northern Hemisphere and to the left in the Southern Hemisphere. Currents flowing from the equator toward the poles tend to be narrow as a result of the Earth’s rotation and carry warm water poleward along the east coasts of adjacent continents. These are called western boundary currents, and they are key contributors to the local climate. The Gulf Stream and the Kuroshio currents in the Northern Hemisphere and the Brazil, Mozambique, and Australian currents in the Southern Hemisphere are such western boundary currents. Currents returning cold water toward the equator tend to be broad and diffuse along the western coasts of adjacent landmasses. These warm western boundary and cold eastern boundary currents affect climate of nearby lands making them warmer or colder than other areas at equivalent latitudes. For example, the warm Gulf Stream makes northern Europe much milder than similar latitudes in northeastern Canada and Greenland. Another example is the cool Peru Current flowing north along the west coast of South America. This cold current limits evaporation in the ocean and contributes to the cool and arid condition of the Atacama Desert in Chile [10].
![By Dr. Michael Pidwirny (see http://www.physicalgeography.net) [Public domain], <a data-cke-saved-href="https://commons.wikimedia.org/wiki/File%3ACorrientes-oceanicas.png" href="https://commons.wikimedia.org/wiki/File%3ACorrientes-oceanicas.png via Wikimedia Commons Warm currents are red, cold currents are blue.](https://geo.libretexts.org/@api/deki/files/33189/Corrientes-oceanicas-300x157.png?revision=1)
Deep Currents
Whether an ocean current moves horizontally or vertically depends on its density. The density of seawater is determined by temperature and salinity, or the amount of salt dissolved in the water. Evaporation and the influx of freshwater from rivers affect salinity and therefore the density of seawater. As the western boundary currents cool at high latitudes and salinity increases due to evaporation and ice formation (recall that ice floats; water is densest just above its freezing point), the cold, denser water sinks to become the ocean’s deep waters. Movement of this deep water is called the thermohaline circulation (thermo refers to temperature, haline refers to salinity). This circulation connects the deep waters of all the world’s oceans. Movement of the Gulf Stream illustrates the beginning of thermohaline circulation. Heat in the warm poleward moving Gulf Stream promotes evaporation which takes heat from the water and as the heat dissipates, the water cools. The resulting water is much colder, saltier, and denser. As the denser water reaches the North Atlantic and Greenland, it begins to sink and becomes a deep-water current. As shown in the illustration below, this worldwide connection between shallow and deep-ocean circulation overturns and mixes the entire world ocean, bringing nutrients to marine life, and is sometimes referred to as the global conveyor belt [11].
![Source: By Robert Simmon, NASA. Minor modifications by Robert A. Rohde also released to the public domain (NASA Earth Observatory) [Public domain], <a data-cke-saved-href="https://commons.wikimedia.org/wiki/File%3AThermohaline_Circulation_2.png" href="https://commons.wikimedia.org/wiki/File%3AThermohaline_Circulation_2.png via Wikimedia Commons In certain areas, the current sinks or rises.](https://geo.libretexts.org/@api/deki/files/33191/Thermohaline_Circulation-300x188.png?revision=1&size=bestfit&width=488&height=306)
Tides
The gravitational effects of the Sun and Moon on the oceans create tides, the rising and lowering of sea level during the day [12]. The Earth rotates daily within the gravity fields of the Moon and Sun. Although the Sun is much larger and its gravitational pull is more powerful, its great distance from Earth means that the gravitational influence of the Moon on tides is dominant. The magnitude of the tide at a given location and the difference between high and low tide (the tidal range) depends primarily on the configuration of the Moon and Sun with respect to the Earth's orbit and rotation. When the Sun, Moon, and Earth line up with each other at full moon or new moon, the tidal range is at a maximum. This is called spring tide. Approximately two weeks later when the Moon and Sun are at right angles with the Earth, the tidal range is lowest. This is called neap tide.

The Earth rotates within a tidal envelope so we experience the rising and ebbing of the tide on a daily basis. Tides are measured at coastal locations and these measurements and tidal predictions based on them are published for those who depend on this information (e.g. this NOAA website) [13]. The rising and falling of the tides (tidal patterns) as experienced at a given shore location are of three types: diurnal, semidiurnal, and mixed.
![Source: By KVDP (Own work) [Public domain], <a data-cke-saved-href="https://commons.wikimedia.org/wiki/File%3ADiurnal_tide_types_map.jpg" href="https://commons.wikimedia.org/wiki/File%3ADiurnal_tide_types_map.jpg via Wikimedia Commons The map shows locations of the different tide types.](https://geo.libretexts.org/@api/deki/files/33194/Diurnal_tide_types_map-300x147.jpg?revision=1&size=bestfit&width=635&height=311)
Diurnal tides go through a complete cycle once in each tidal day. Keeping in mind that the Moon orbits around the Earth, a tidal day is the amount of time for the Moon to align with a point on the Earth as the Earth rotates, which is slightly longer than 24 hours. Semidiurnal tides go through two complete cycles in each tidal day—approximately 24 hours and 50 minutes, with the tidal range typically varying in each cycle. Mixed tides are a combination of diurnal and semidiurnal patterns and show two tidal cycles per tidal day, but the relative amplitudes of each cycle and their highs and lows vary during the tidal month. For example, there is a high high-tide and a high low-tide. The next day, there is a low high-tide and a low low-tide.
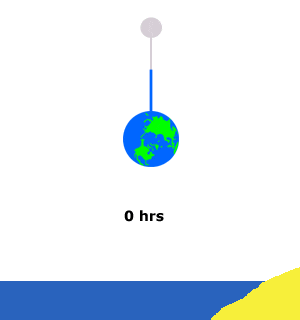
The pattern at a given shore location and the times of arrival of tidal phases are complicated and determined by the bathymetry (depth) of the ocean basins and continental obstacles in the way of the tidal envelope within which the Earth rotates. Local tidal experts make 48-hour tidal forecasts using tidal charts based on daily observations, as can be seen in the chart of different tide types. Typical tidal ranges are on the order of 1 meter (3 feet). Extreme tidal ranges occur where the tidal wave enters a narrow restrictive zone that funnels the tidal energy. An example is the English Channel between Great Britain and the European continent where tidal ranges of 7 to 9.5 meters (25 to 32 feet) have been observed. The Earth’s highest tidal ranges occur at the Bay of Fundy, the funnel-like bay between Nova Scotia and New Brunswick, Canada, where the average range is nearly 12 meters (40 feet) and the extreme range of around 18 meters (60 feet). At these locations of extreme tidal range, a person who ventures out onto the seafloor exposed during ebb tide may not be able to outrun the advancing water during flood tide. Additional information on tides can be found at this NOAA website.

References
9. Colling, A. Ocean Circulation. (Butterworth-Heinemann, 2001).
10. Munk, W. H. On the wind-driven ocean circulation. J. Meteorol. 7, 80–93 (1950).
11. Stommel, H. & Arons, A. B. On the abyssal circulation of the world ocean—I. Stationary planetary flow patterns on a sphere. Deep Sea Research (1953) 6, 140–154 (1959).
12. Stewart, R. H. Introduction to physical oceanography. (Texas A & M University Texas, 2008).
13. Schwiderski, E. W. On charting global ocean tides. Rev. Geophys. 18, 243–268 (1980).