17.1: Front Matter
- Page ID
- 14864
\( \newcommand{\vecs}[1]{\overset { \scriptstyle \rightharpoonup} {\mathbf{#1}} } \)
\( \newcommand{\vecd}[1]{\overset{-\!-\!\rightharpoonup}{\vphantom{a}\smash {#1}}} \)
\( \newcommand{\id}{\mathrm{id}}\) \( \newcommand{\Span}{\mathrm{span}}\)
( \newcommand{\kernel}{\mathrm{null}\,}\) \( \newcommand{\range}{\mathrm{range}\,}\)
\( \newcommand{\RealPart}{\mathrm{Re}}\) \( \newcommand{\ImaginaryPart}{\mathrm{Im}}\)
\( \newcommand{\Argument}{\mathrm{Arg}}\) \( \newcommand{\norm}[1]{\| #1 \|}\)
\( \newcommand{\inner}[2]{\langle #1, #2 \rangle}\)
\( \newcommand{\Span}{\mathrm{span}}\)
\( \newcommand{\id}{\mathrm{id}}\)
\( \newcommand{\Span}{\mathrm{span}}\)
\( \newcommand{\kernel}{\mathrm{null}\,}\)
\( \newcommand{\range}{\mathrm{range}\,}\)
\( \newcommand{\RealPart}{\mathrm{Re}}\)
\( \newcommand{\ImaginaryPart}{\mathrm{Im}}\)
\( \newcommand{\Argument}{\mathrm{Arg}}\)
\( \newcommand{\norm}[1]{\| #1 \|}\)
\( \newcommand{\inner}[2]{\langle #1, #2 \rangle}\)
\( \newcommand{\Span}{\mathrm{span}}\) \( \newcommand{\AA}{\unicode[.8,0]{x212B}}\)
\( \newcommand{\vectorA}[1]{\vec{#1}} % arrow\)
\( \newcommand{\vectorAt}[1]{\vec{\text{#1}}} % arrow\)
\( \newcommand{\vectorB}[1]{\overset { \scriptstyle \rightharpoonup} {\mathbf{#1}} } \)
\( \newcommand{\vectorC}[1]{\textbf{#1}} \)
\( \newcommand{\vectorD}[1]{\overrightarrow{#1}} \)
\( \newcommand{\vectorDt}[1]{\overrightarrow{\text{#1}}} \)
\( \newcommand{\vectE}[1]{\overset{-\!-\!\rightharpoonup}{\vphantom{a}\smash{\mathbf {#1}}}} \)
\( \newcommand{\vecs}[1]{\overset { \scriptstyle \rightharpoonup} {\mathbf{#1}} } \)
\( \newcommand{\vecd}[1]{\overset{-\!-\!\rightharpoonup}{\vphantom{a}\smash {#1}}} \)
\(\newcommand{\avec}{\mathbf a}\) \(\newcommand{\bvec}{\mathbf b}\) \(\newcommand{\cvec}{\mathbf c}\) \(\newcommand{\dvec}{\mathbf d}\) \(\newcommand{\dtil}{\widetilde{\mathbf d}}\) \(\newcommand{\evec}{\mathbf e}\) \(\newcommand{\fvec}{\mathbf f}\) \(\newcommand{\nvec}{\mathbf n}\) \(\newcommand{\pvec}{\mathbf p}\) \(\newcommand{\qvec}{\mathbf q}\) \(\newcommand{\svec}{\mathbf s}\) \(\newcommand{\tvec}{\mathbf t}\) \(\newcommand{\uvec}{\mathbf u}\) \(\newcommand{\vvec}{\mathbf v}\) \(\newcommand{\wvec}{\mathbf w}\) \(\newcommand{\xvec}{\mathbf x}\) \(\newcommand{\yvec}{\mathbf y}\) \(\newcommand{\zvec}{\mathbf z}\) \(\newcommand{\rvec}{\mathbf r}\) \(\newcommand{\mvec}{\mathbf m}\) \(\newcommand{\zerovec}{\mathbf 0}\) \(\newcommand{\onevec}{\mathbf 1}\) \(\newcommand{\real}{\mathbb R}\) \(\newcommand{\twovec}[2]{\left[\begin{array}{r}#1 \\ #2 \end{array}\right]}\) \(\newcommand{\ctwovec}[2]{\left[\begin{array}{c}#1 \\ #2 \end{array}\right]}\) \(\newcommand{\threevec}[3]{\left[\begin{array}{r}#1 \\ #2 \\ #3 \end{array}\right]}\) \(\newcommand{\cthreevec}[3]{\left[\begin{array}{c}#1 \\ #2 \\ #3 \end{array}\right]}\) \(\newcommand{\fourvec}[4]{\left[\begin{array}{r}#1 \\ #2 \\ #3 \\ #4 \end{array}\right]}\) \(\newcommand{\cfourvec}[4]{\left[\begin{array}{c}#1 \\ #2 \\ #3 \\ #4 \end{array}\right]}\) \(\newcommand{\fivevec}[5]{\left[\begin{array}{r}#1 \\ #2 \\ #3 \\ #4 \\ #5 \\ \end{array}\right]}\) \(\newcommand{\cfivevec}[5]{\left[\begin{array}{c}#1 \\ #2 \\ #3 \\ #4 \\ #5 \\ \end{array}\right]}\) \(\newcommand{\mattwo}[4]{\left[\begin{array}{rr}#1 \amp #2 \\ #3 \amp #4 \\ \end{array}\right]}\) \(\newcommand{\laspan}[1]{\text{Span}\{#1\}}\) \(\newcommand{\bcal}{\cal B}\) \(\newcommand{\ccal}{\cal C}\) \(\newcommand{\scal}{\cal S}\) \(\newcommand{\wcal}{\cal W}\) \(\newcommand{\ecal}{\cal E}\) \(\newcommand{\coords}[2]{\left\{#1\right\}_{#2}}\) \(\newcommand{\gray}[1]{\color{gray}{#1}}\) \(\newcommand{\lgray}[1]{\color{lightgray}{#1}}\) \(\newcommand{\rank}{\operatorname{rank}}\) \(\newcommand{\row}{\text{Row}}\) \(\newcommand{\col}{\text{Col}}\) \(\renewcommand{\row}{\text{Row}}\) \(\newcommand{\nul}{\text{Nul}}\) \(\newcommand{\var}{\text{Var}}\) \(\newcommand{\corr}{\text{corr}}\) \(\newcommand{\len}[1]{\left|#1\right|}\) \(\newcommand{\bbar}{\overline{\bvec}}\) \(\newcommand{\bhat}{\widehat{\bvec}}\) \(\newcommand{\bperp}{\bvec^\perp}\) \(\newcommand{\xhat}{\widehat{\xvec}}\) \(\newcommand{\vhat}{\widehat{\vvec}}\) \(\newcommand{\uhat}{\widehat{\uvec}}\) \(\newcommand{\what}{\widehat{\wvec}}\) \(\newcommand{\Sighat}{\widehat{\Sigma}}\) \(\newcommand{\lt}{<}\) \(\newcommand{\gt}{>}\) \(\newcommand{\amp}{&}\) \(\definecolor{fillinmathshade}{gray}{0.9}\)Our Oceans
Oceans cover 71% of Earth’s surface and hold 97% of Earth’s water, yet nearly 95% of that ocean is completely unexplored. The entire ocean floor has been mapped, but only to a resolution of 3 miles: this means that we can only see features larger than 3 miles wide. We have better maps of the surface of Mars and the moon than we do the bottom of the ocean. We know very, very little about most of the ocean, particularly for the middle and deeper parts far away from the coasts. Why? It is a challenging place to work! In many ways, it is easier to put a person into space than it is to send a person down to the bottom of the ocean. It is dark, cold, and the pressure exerted by the water above is enormous, equivalent to one person trying to support 50 jumbo jets. However, the water that the oceans contain is critical to plate tectonics, volcanism, and of course, life on Earth. The ocean floor is covered with an average of 13,000 feet of water and is pitch black below a few thousand feet or so; it is not easy to discover what is down there. We know a lot more about the oceans than we used to, but there is still a great deal more to discover.
Earth has had oceans for a very long time, dating back to the point where the surface had cooled enough to allow liquid water to condensate, only a few hundred million years after Earth’s formation. At that time there were no continental rocks, so Earth’s water was likely spread out over the surface in one giant (but relatively shallow) ocean.
A person who studies the oceans and coastal processes may have many titles including oceanographer, coastal geomorphologist, sedimentologist, or climate scientist. Like many other geoscientists, working with other disciplines is common, with a heavy influence from both math and technology. Many are employed by universities where they teach and/or do research, and state and federal agencies, including geological surveys, like the California Geological Survey or United State Geological Survey (USGS). Additional career pathways include environmental policy and legislation and consulting, via the private sector or state and federal agencies. Many of these career options require a college degree and postgraduate work. If this pathway is of interest to you, talk to your geology instructor for advice. We recommend completing as many math and science courses as possible. Also, visit National Parks, CA State Parks, museums, gem & mineral shows, or join a local rock and mineral club. Typically, natural history museums will have wonderful displays of rocks, including those from your local region. Here in California, there are a number of large collections, including the San Diego Natural History Museum, Natural History Museum of Los Angeles County, Santa Barbara Museum of Natural History, and Kimball Natural History Museum. Many colleges and universities also have their own collections/museums.
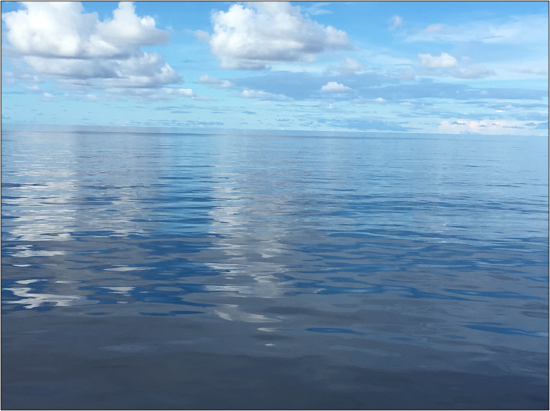
Topography of the Sea Floor
We examined the topography of the sea floor from the perspective of plate tectonics and in sedimentary rocks but here we are going to take another look at bathymetry from an oceanographic perspective. Bathymetry is the study of submarine topography. The topography of the ocean floor is shown in Figure 17.2. Notice the variety of blue colors: light blue indicates shallow ocean water whereas darker blues indicate deeper ocean waters.
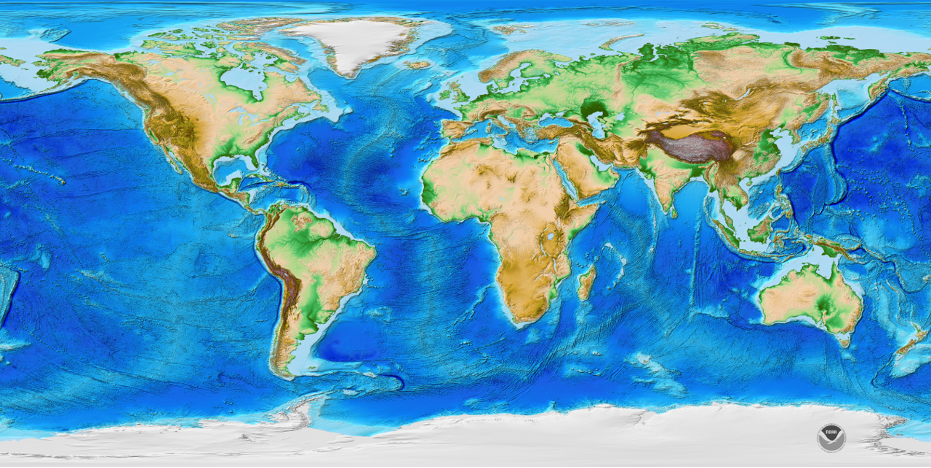
A topographic profile of the Pacific Ocean floor between Japan and the Pacific Northwest is shown in Figure 17.3. This diagram has a significant amount of vertical exaggeration (the vertical scale overemphasizes the height of the features relative to their width), but it captures the varied topography of the seafloor well. From the deepest trenches (up to 7 miles deep at the Mariana Trench) to the highest mountains (Mauna Kea on the Big Island of Hawaii is taller than Mount Everest, when measured from its base to its summit), the floor of the Pacific is not the flat basin we once envisioned. The vast sediment-covered abyssal plains of the oceans, however, are much flatter than any similar-sized areas on the continents and cover more than 50% of the Earth’s surface (Figure 17.4). These extremely deep (over 10,000 feet) and flat areas of the sea-floor are interrupted by massive underwater mountain chains, seamounts, or MORs (Figure 17.3). Other major topographic features in the oceans include the continental shelves, slopes, and rises that surround the continental crust.
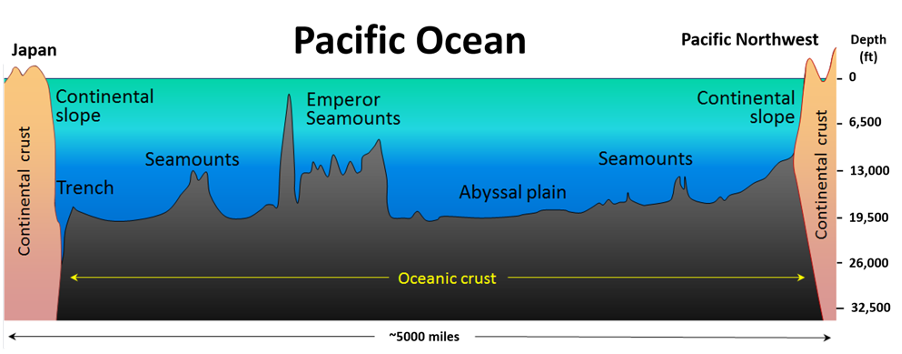
Starting from land, a trip across an ocean basin along the seafloor would begin with crossing the continental shelf (Figure 17.4). The continental shelf is an area of relatively shallow water, usually less than a few hundred feet deep, that surrounds a continent. It is narrow or nearly nonexistent in some places; in others, it can extend for hundreds of miles. On the East and Gulf Coasts, where there are passive margins (no plate boundaries), the continental shelf averages around 85 miles wide. On the West Coast, where there is an active margin, the shelf is less than half as wide. Due to the abundance of light and nutrients from upwelling and runoff, the waters along the continental shelf are usually biologically productive.
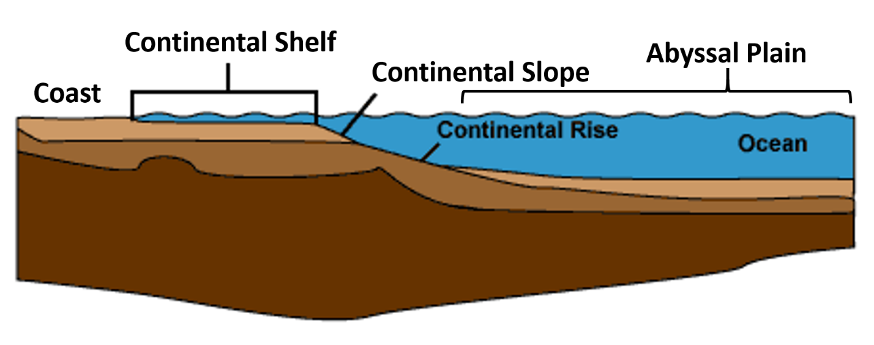
At the edge of the shelf is the boundary known as the continental slope, which separates the shelf from the continental rise (Figure 17.4). The continental rise is very slightly angled, between 0.5 degrees and 1.0 degrees. Deposition of sediments at the mouth of submarine canyons carved into the shelf and slope may form enormous fan-shaped accumulations called submarine fans on both the continental slope and continental rise.
Waves
Waves form on the ocean and on lakes because energy from wind is transferred to the water. The stronger the wind, the longer it blows; the larger the area of water over which it blows, referred to as the wind’s fetch, the larger the waves are likely to be. The important parameters of a wave are 1) its wavelength, the horizontal distance between two crests or two troughs, 2) its amplitude, half the vertical distance between a trough and a crest, and 3) its velocity, the speed at which wave crests move across the water. (Figure 17.5).
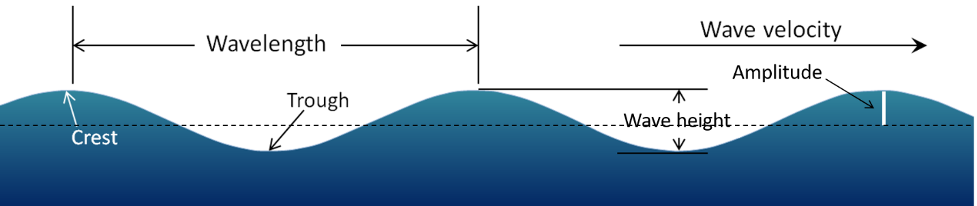
As a wave moves across the surface of the water, the water itself mostly just moves up and down and only moves a small amount in the direction of wave motion. As this happens, a point on the water surface circumscribes a circle with a diameter that is equal to the wave amplitude (Figure 17.6). This motion is also transmitted to the water underneath, and the water is disturbed by a wave to a depth of approximately one-half of the wavelength. Wave motion is illustrated quite clearly on the Wikipedia “Wind wave” site. If you look carefully at that animation and focus on the small white dots in the water, you should be able to see how the amount that they move decreases with depth.
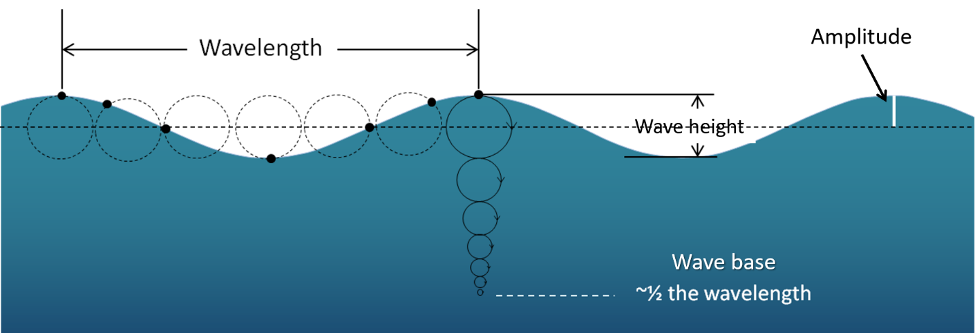
The one-half wavelength depth of disturbance of the water beneath a wave is known as the wave base. Since ocean waves rarely have wavelengths greater than 650 feet, and the open ocean is several thousand feet deep, the wave base does not normally interact with the bottom of the ocean. However, as waves approach the much shallower water near the shore, they start to “feel” the bottom and are affected by this interaction. The wave “orbits” are both flattened and slowed by dragging, with the implication that the wave amplitude (height) increases and the wavelength decreases (the waves become much steeper). The ultimate result of this is that the waves lean forward, and eventually break.
Waves normally approach the shore at an angle, which means that one part of the wave feels the bottom sooner than the rest of it; the part that feels the bottom first will slow down first. Even though they bend and become nearly parallel to shore, most waves still reach the shore at a small angle, and as each one arrives, it pushes water along the shore, creating what is known as a longshore current within the surf zone. Another important effect of waves reaching the shore at an angle is that when they wash up onto the beach, they do so at an angle, but when that same wave water flows back down the beach, it moves straight down the slope of the beach. The upward-moving water, known as the swash, pushes sediment particles along the beach, while the downward-moving water, the backwash, brings them straight back. With every wave that washes up and then down the beach, particles of sediment are moved along the beach in a zigzag pattern. The combined effects of sediment transport within the surf zone by the longshore current and sediment movement along the beach by swash and backwash is known as longshore drift (Figure 17.7). Longshore drift moves a tremendous amount of sediment along coasts of oceans and large lakes around the world and it is responsible for creating a variety of depositional features.
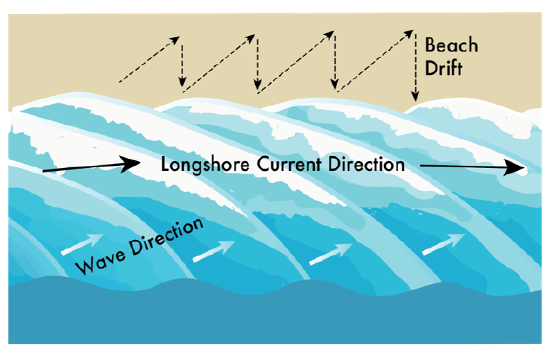
A rip current is another type of current that develops in the nearshore area and has the effect of returning water that has been pushed up to the shore by incoming waves. Rip currents flow straight out from the shore and are fed by the longshore currents. They die out quickly just outside the surf zone but can be dangerous to swimmers who get caught in them. If part of a beach does not have a strong unidirectional longshore current, the rip currents may be fed by longshore currents going in both directions.
Tides are very long-wavelength but low-amplitude waves on the ocean surface that are caused by variations in the gravitational effects of the Sun and Moon. Tide amplitudes in shoreline areas vary quite dramatically from place to place. As the tides rise and fall they push and pull a large volume of water in and out of bays and inlets and around islands. They do not have as significant an impact on coastal erosion and deposition as wind waves do, but they have an important influence on the formation of features within the intertidal zone.
Erosional and Depositional Landforms
Some coastal areas are dominated by erosion, like California and the rest of the US Pacific Coast; other coastlines are dominated by deposition, like the Atlantic and Gulf Coasts (Figure 17.8). On almost all coasts, however, both deposition and erosion are happening to varying degrees in different places. For this introductory course, we will oversimplify the North American coastlines to illustrate basic concepts: the West Coast is emergent and therefore erosional, while the East and Gulf Coasts are submergent and therefore depositional. The real world is obviously more complex, and it is possible to find all landforms on both coastlines.
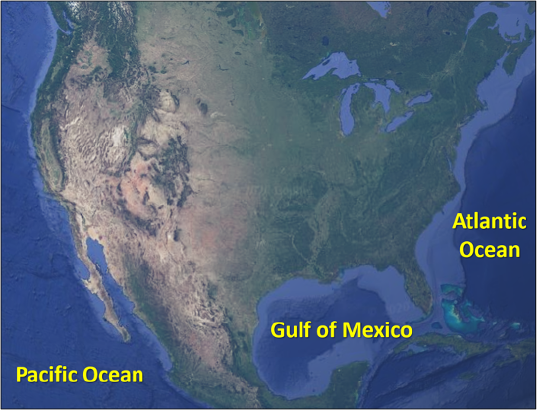
Erosional Landforms
A key factor in determining if a coast is dominated by erosion or deposition is its history of tectonic activity. An emergent coastline has been exposed by the relative fall in sea level by either isostasy, eustasy, or tectonic uplift. In general, California’s coastline is relatively active tectonically; uplift for tens of millions of years has resulted in a local “fall” in sea-level. Our emergent coastlines are typically dominated by erosional features, with rocky shores, narrow beaches and continental shelves.
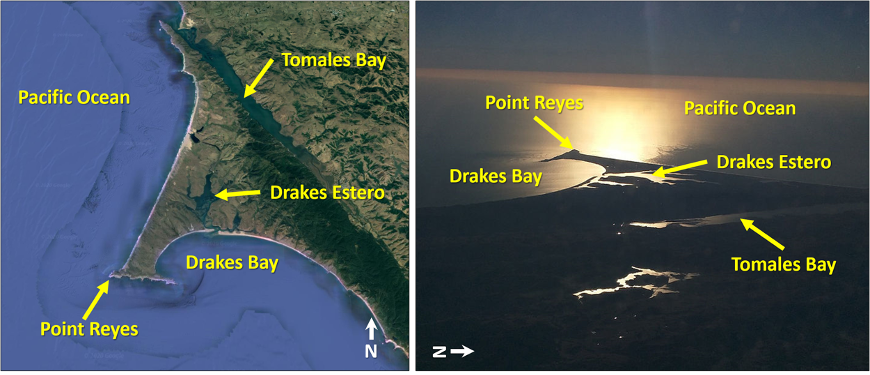
Several unique erosional features commonly form on these rocky shores. When waves approach an irregular shore, they are slowed down to varying degrees, depending on differences in the water depth, and as they slow, they are bent or refracted. Because of refraction, the energy of the waves, which moves perpendicular to the wave crests, is focused on the headlands (Figure 17.9).
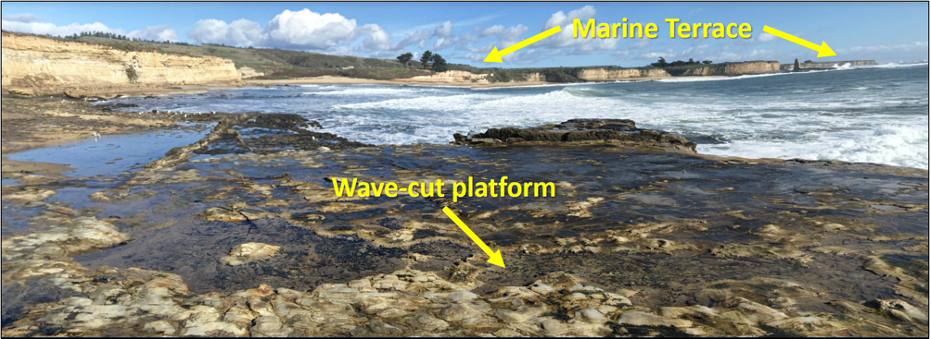
Wave erosion is greatest in the surf zone, where the wave base strongly affects the seafloor and waves break. The result is a narrow flat surface known as a wave-cut platform or wave-cut terrace, found at the base of a sea cliff or along the shoreline (Figure 17.10). Read more about marine terraces from the USGS - Fact Sheet, Landscapes from the Waves - Marine Terraces of California.
More resistant rock that does not erode completely during the formation of a wave-cut platform often remains behind to form a sea stack. These sea stacks are typically steep, vertical columns of rock formed over time. Sea arches and sea caves, the results of sequential erosion of promontories and headlands, may eventually collapse and form stacks (Figure 17.11).
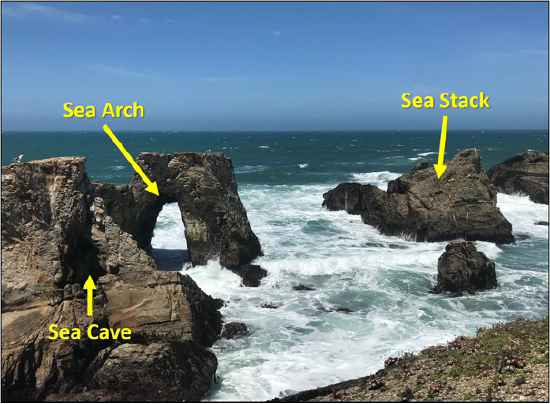
Depositional Landforms
Recall that emergent coastlines result from a relative fall in sea level. Accordingly, a submergent coastline has been covered or inundated by the sea as a result of a relative rise in sea level from either isostasy or eustasy. The US Atlantic and Gulf Coasts are passive margins and have not seen much tectonic activity during the last hundred million years. Generally, submergent coastlines are dominated by deposition, although erosion will still occur, and are characterized by sandy beaches and wide continental shelves.
The evolution of sandy depositional features on seacoasts is primarily influenced by waves and currents, especially longshore currents. As sediment is transported along a shore, it is either deposited on beaches, or creates another depositional feature. A beach is a landform along a body of water that consists of loose sediments, which range in size and composition but are typically derived from the local bedrock, shells or coral. A spit, is an elongated sandy deposit, similar to a beach, that extends out into open water in the direction of a longshore current (Figure 17.12). Should the spit begin to grow backwards and curve, it is referred to as a hook. A spit that extends across a bay to the extent of closing, or almost closing it off, is known as a baymouth bar. Most bays have rivers flowing into them, and since this water must get out, it is rare that a baymouth bar will completely close the entrance to a bay. In areas with near-shore islands and sufficient sediment transport, a tombolo and tied-island may develop. In areas where coastal sediments are abundant and coastal relief is low, it is also common for barrier islands to develop. These islands are composed entirely of sand and have a distinct elongated shape; typically they develop only a few miles from the mainland (Figure 17.12).
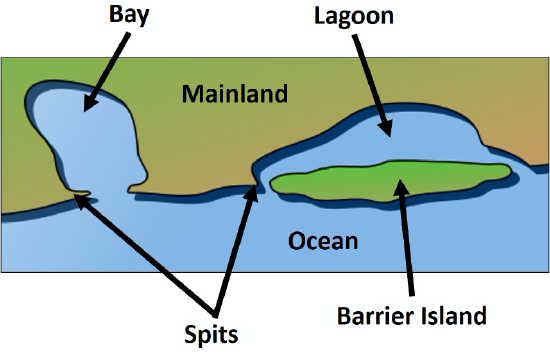
Human Interface with Shorelines
There are various modifications that we make to influence shoreline processes for our own purposes. Sometimes these changes are effective and appear to be beneficial, although in most cases there are unintended negative consequences that we do not recognize until much later.
Occasionally beaches are armored with riprap and concrete blocks to limit the natural erosion that threatens the properties at the shore (Figure 17.13). The unintended effect of this installation will be to deprive the area of sediment. If the armor remains in place for several decades, there is a significant risk that the depositional landforms will start to erode. This could also affect the biology of the area, including many of the organisms that use that area as their habitat, and people who use the area for recreation.
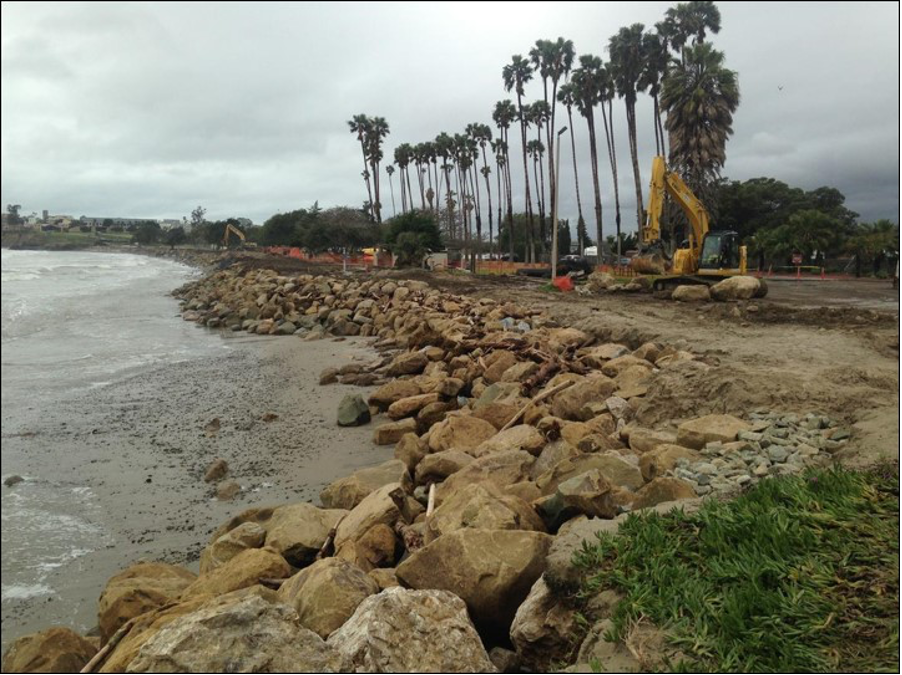
Seawalls also limit erosion and can be useful amenities for the public; however, they too have geological and ecological costs. Seawalls affect the behavior of waves and longshore currents, sometimes with negative results. When a shoreline is “hardened” in this way, important marine habitat is lost and sediment production is reduced, which can affect beaches elsewhere.
Breakwaters are structures that run parallel to the shore (Figure 17.14). Typically, these have acted as islands and sand is deposited in the low-energy water behind them, similar to how a tombolo may form. Negative impacts are not yet well understood but likely involve loss of marine animal habitat.
Groins are structures constructed perpendicular to the beach (Figure 17.14). They have an effect that is similar to breakwaters, while trapping sediment by slowing the longshore current.
Most of the sediment that forms beaches along our coasts comes from rivers, so if we want to take care of beaches, we must take care of rivers. When a river is dammed, its sediment load is deposited in the resulting reservoir, and while the reservoir is filling up that sediment cannot get to the sea. During this time, beaches and other depositional landforms within miles of the river’s mouth are at risk of erosion as longshore currents displace sediment that is not replenished by the river.
For more on our nation's changing coastlines, read this USGS Fact Sheet - Shoreline Change Research.
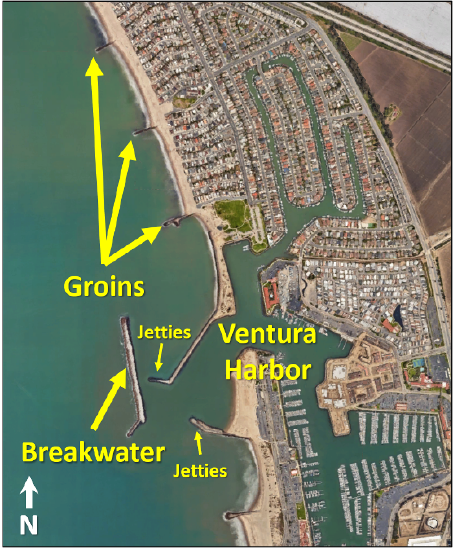
Attributions
- Figure 17.1: “Doldrums of the Pacific” (CC-BY 4.0; Chloe Branciforte, own work)
- Figure 17.2: “ETOPO1 1 Arc-Minute Global Relief Model” (Public Domain; C. Amante and B.W. Eakins via NOAA)
- Figure 17.3: “Generalized Topography of the Pacific Ocean” (CC-BY 4.0; Steven Earle via OpenText)
- Figure 17.4: Derivative of “Continental Shelf” (Public Domain; Interiot via Wikimedia Commons) by Chloe Branciforte
- Figure 17.5: “The Parameters of Water Waves” (CC-BY 4.0; Steven Earle via OpenText)
- Figure 17.6: “Wave Motion” (CC-BY 4.0; Steven Earle via OpenText)
- Figure 17.7: “Longshore Current” (CC-BY 4.0; Emily Haddad, own work)
- Figure 17.8: “Water Bodies” (CC-BY 4.0; Chloe Branciforte via Google Earth, own work)
- Figure 17.9: Derivative of “Point Reyes and Drakes Bay” (CC-BY-SA 4.0; DickLyon via Wikimedia Commons) by Chloe Branciforte and Google Earth
- Figure 17.10: “Marine Terrace and Platform” (CC-BY 4.0; Chloe Branciforte via Google Earth, own work)
- Figure 17.11: Derivative of “Point Arena-Stornetta” (Public Domain; Samantha Storms via BLM)
- Figure 17.12: Derivative of “Accreting Coast Image6” (Public Domain; Surachit via Wikipedia)
- Figure 17.13: “Armoring the Shore at Goleta Beach” (Public Domain; Daniel Hoover via USGS)
- Figure 17.14: “Ventura Harbor” (CC-BY 4.0; Chloe Branciforte via Google Earth, own work)