7.1: Front Matter
- Page ID
- 14551
What Is the Rock Cycle?
The rock cycle is a circular diagram that demonstrates the process by which rocks are continually recycled throughout geologic time. All rocks can be classified into one of three groups: igneous, sedimentary, or metamorphic. This rock classification is based on the origin of each of these rock types, or the processes that form the rock. The Rock Cycle (Figure 7.1), represents the processes that produce each of the rock types. Igneous rocks are produced through the melting of rock and eventual cooling of lava or magma. As cooling occurs, crystals may form. Sedimentary rocks are produced via the processes of weathering (the chemical and physical breakdown of material at Earth’s surface that produces sediments), erosion (the movement and deposition of sediments), and lithification (the compaction and cementation of sediments). Metamorphic rocks are produced via the agents of change, primarily heat and pressure, which typically result in the recrystallization of minerals. These processes will be explored in greater detail in each of their respective chapters.
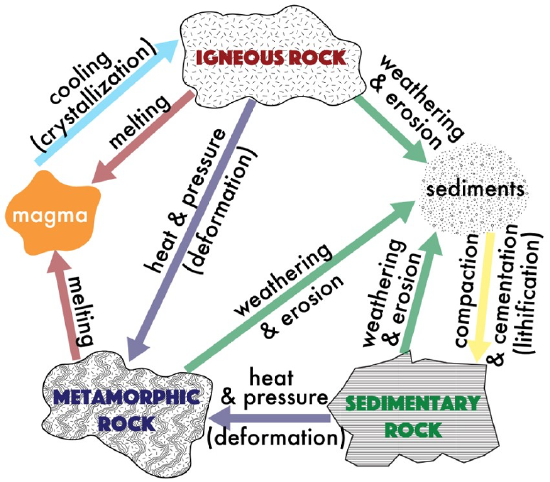
Igneous Rocks
Igneous rocks are the only rocks that form from a liquid (or molten) state. All igneous rocks start out as molten material (magma or lava), which must harden to form a rock. Igneous rocks differ from one another primarily due to: 1) the composition of the molten material from which the rock is derived, and 2) the cooling process of the molten material that formed the rock. These two parameters define the classification of igneous rocks, which are simplified into the two terms: composition and texture. Igneous rock composition refers to what is in the rock (the chemical composition or the minerals that are present), and the word texture refers to the features that we see in the rock, such as the mineral sizes or the presence of glass, fragmented material, or vesicles (holes) in the igneous rock.
A person who studies igneous rocks is referred to as an igneous petrologist. A petrologist is a geoscientist that studies rocks and the conditions under which they form. An igneous petrologist is a geoscientist who specializes in igneous rocks and their conditions of formation. Like many other geoscientists, working with other disciplines is common, with a heavy influence from both math and technology. Many are employed by universities where they teach and/or do research, and at state and federal agencies, including geological surveys, like the California Geological Survey or United States Geological Survey (USGS). Additional career pathways are available in the private sector including mining and natural resource extraction. Many of these career options require a college degree and postgraduate work. If you are interested, talk to your geology instructor for advice. We recommend completing as many math and science courses as possible (chemistry is incredibly important for mineralogy). Also, visit National Parks, CA State Parks, museums, gem & mineral shows, or join a local rock and mineral club. Typically, natural history museums will have wonderful displays of rocks, including those from your local region. Here in California, there are a number of large collections, including the San Diego Natural History Museum, Natural History Museum of Los Angeles County, Santa Barbara Museum of Natural History, and Kimball Natural History Museum. Many colleges and universities also have their own collections/museums.
Melting
What Is a Melt?
A “melt” refers to molten rock, typically formed at high temperatures (~2000°F/1090°C). Melts are characterized by where they are located. Lava exists at or very near the surface of the Earth, whereas magma forms at depth within the crust and lithosphere. Although the term “melt” indicates a rock that is completely molten (i.e. a liquid), most melts actually contain all three states of matter. The liquid component is the actual molten rock. The solid components are minerals that have crystallized or glasses that have formed. Lastly, the gaseous components are dissolved within the melt, like carbon dioxide, water vapor, and others. These gases will be an important driver in volcanic eruptions.
Where Do Melts Form?
There are a number of myths surrounding melts. The first often perpetuated myth is that melts come from the outer core. This is FALSE. A melt can only originate in the mantle or lithosphere. The second often perpetuated myth is that melts exist everywhere beneath the crust. This is also FALSE. Melts only form in specific tectonic settings, including continental volcanic arcs and volcanic island arcs (subduction zones), hot spots, and divergent boundaries (mid-ocean ridges and rifts).
How Do Rocks Melt?
Melting is an interplay between temperature and pressure. Under normal or low pressures, like on the surface of the Earth, you need only 1) add heat, 2) meet or exceed the melting temperature of the object you are trying to melt, and 3) a melt will be produced. For example, chocolate has a relatively low melting temperature, around 90°F, while our body temperature is around 98°F; this is why chocolate will melt in your hand (and in your mouth!). Earth’s “body” temperature runs much hotter, and temperatures increase with depth moving towards the core; however, pressure also increases with depth. Increasing pressure counteracts increasing temperature, preventing the breaking of mineral bonds and disruption of crystal lattices that are required to melt rocks. In Figure 7.2, the red lines represent the geothermal gradient, which tracks how temperature increases with depth; the gray areas on the charts represent the temperature and pressure conditions under which rock remains solid, whereas the pink regions represent the temperature and pressure conditions under which rock will melt into liquid. Notice how Earth is not molten throughout; instead melts typically only exist within the crust and upper lithosphere, usually fewer than 50 miles (80 km) from the surface, and form only in unique tectonic settings where specific conditions are met (i.e. where the geothermal gradient crosses the solidus curve).
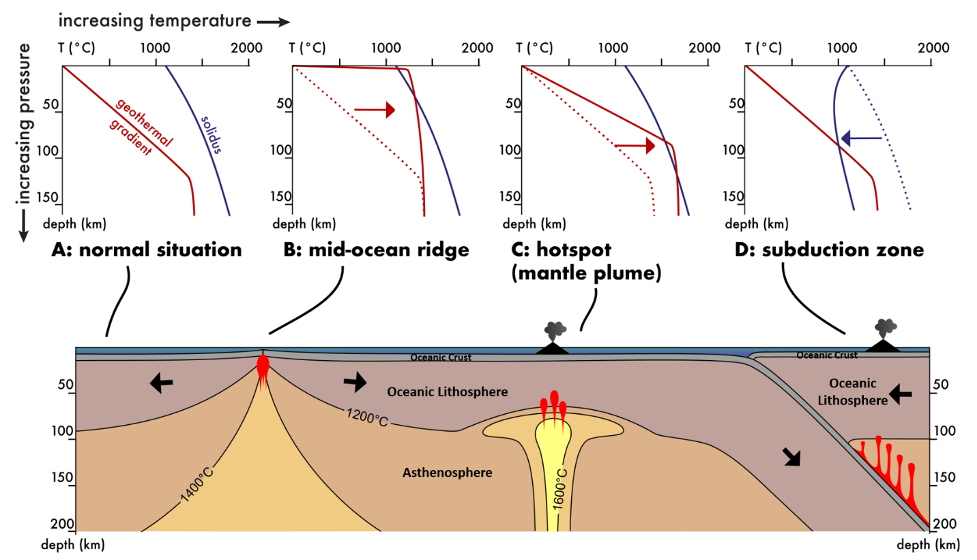
One way to surpass the melting temperature of a rock and create a melt is through a process called decompression melting (Figure 7.3). This process relieves pressures on the rock, which shifts the geothermal gradient. Decompression melting occurs at divergent boundaries (MORs and rifts) and hot spots (like Hawaii) (Figure 7.2B and C). Here, rock is decompressed at a constant temperature. Convection brings hot mantle from depth and moves it towards the surface. The mantle material stays hotter than the surrounding rocks and, as pressure is relieved, melt is produced. This is why basalt commonly forms at MORs and at hot spots below oceanic plates.
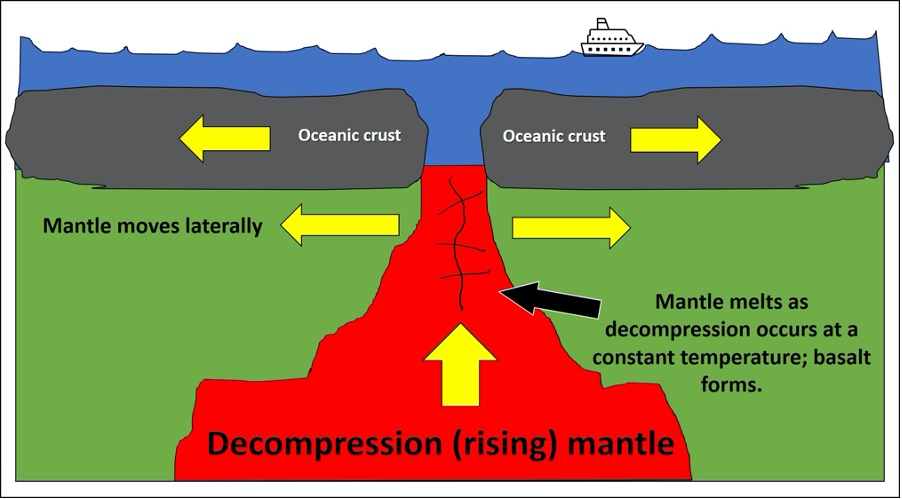
Earth has additional processes at play, which help to lower the melting temperature of rock. Typically, this occurs through the addition of volatiles, in a process called flux melting (Figure 7.4). At subduction zones, hydrated minerals in the oceanic crust release water as they subduct and heat up. This addition of water into the mantle rock causes the mantle to melt at a lower temperature than its normal melting temperature (Figure 7.2D). This process is similar to the use of salt on roadways during the winter months; the salt causes the ice to melt at a lower temperature and helps reduce ice development on roadways. For rock in subduction zones, water behaves like the salt.
Rock can also melt through conduction, a simple way to transfer heat. Melt produced through decompression or flux melting is buoyant and will begin to rise toward Earth's surface. As the rising melt interacts with lithospheric rock, it may transfer enough heat to the surrounding rock to melt it. This is why volcanic arcs (both continental and island) form at subduction zones.
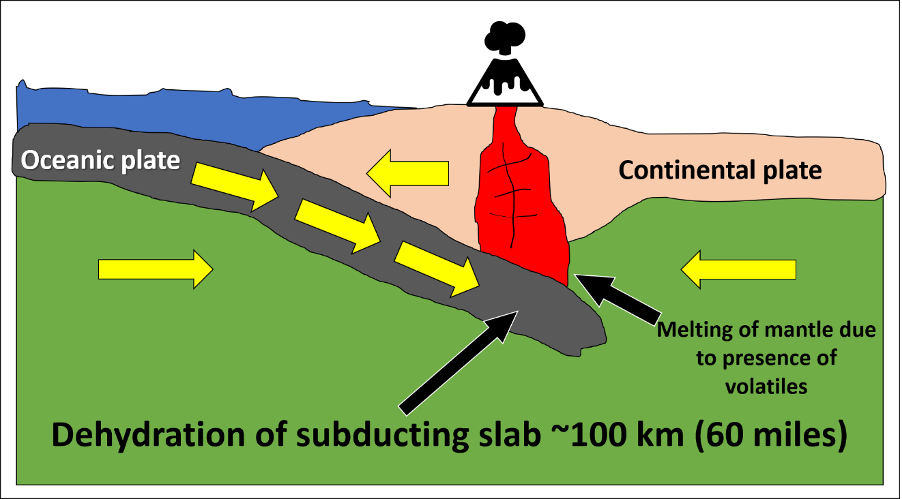
Composition
Composition of Magma
Remember that before an igneous rock can form, there must be molten material known as magma or lava. Most rocks (with very few exceptions) contain minerals that are crystalline solids composed of the chemical elements. From Chapter 3, recall that the most common minerals belong to a group known as the silicate minerals, which are abundant in the rocks of the lithosphere and mantle. Magma that forms from melt in these layers of the Earth also contain these same silicate minerals. All minerals, not just the silicates, are characterized by a certain set of conditions, such as temperature, at which they can melt. Recall that rocks are an aggregate or mixture of minerals. Oftentimes this can result in only some of the minerals in a rock melting, while others stay solid. Temperature conditions are important, as only minerals that melt at “lower” temperatures (590°C/1100°F) may experience melting, whereas the temperature would have to increase (for example, to 1150°C/2100°F) in order for other minerals to also melt. At these higher temperatures, even the lower temperature minerals are still melting and thus adding their chemical components to the magma generated. Even if the same types of rocks are melting, different magma compositions are generated by melting at different temperatures; we refer to this as magma evolution.
Magma is more buoyant than the source rock that generated it, and will eventually start to rise upward through the Earth’s lithosphere. This separation of the magma from the source region will result in new thermal conditions. The magma moves away from the heated portion of the lithosphere and encounters cooler rocks, which results in the magma also cooling. As with melting, minerals also have a certain set of conditions at which they form, or crystallize, from a cooling melt. You would be right in thinking that the sequence of mineral crystallization is the opposite sequence of crystal melting. The sequence of mineral formation from magma was experimentally determined by Norman L. Bowen in the early 1900’s, and the now famous Bowen’s Reaction Series appears in countless textbooks and lab manuals (including this one!; Figure 7.5).
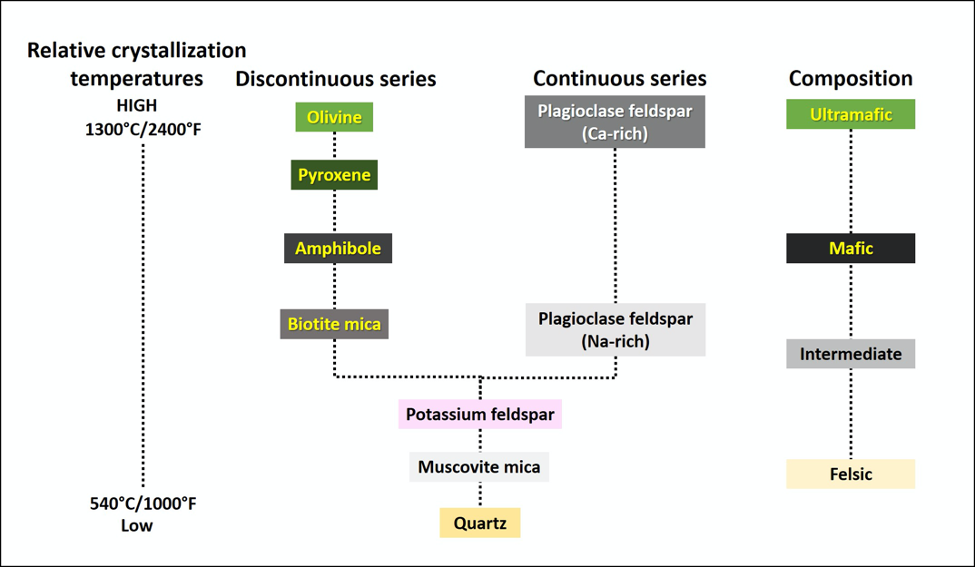
This “reaction series” refers to the chemical reactions that form the minerals, through chemical bonding of elements within the magma, in a sequence that is based on falling magma temperatures. Close examination shows that the first mineral to crystallize in a cooling magma of ultramafic composition is olivine. Once temperatures fall below a specific range, olivine crystals will no longer form; instead, other minerals such as pyroxene will start to crystallize (a small interval of temperatures exists where both olivine and pyroxene can crystallize). Minerals that form in cooling magma are called crystals. As these crystals are forming, they are removing chemical elements from the magma. For example, olivine crystals take magnesium (Mg) and iron (Fe) from the magma and incorporate them into their crystal structure. This behavior of mineral crystals to take certain chemical elements into their structure, while excluding other elements, means that the composition of the magma must be changing as crystals are forming. This is why magmas continuously evolve: their chemistry is always changing.
There can be more than one mineral type crystallizing within the cooling magma. The minerals on the left side of Bowen’s Reaction Series are referred to as a discontinuous series, as these minerals (olivine, pyroxene, amphibole, and biotite) all remove the iron (Fe), magnesium (Mg), and manganese (Mn) from the magma during crystallization, but do so at certain temperature ranges. These iron- and magnesium-rich minerals are referred to as the ferromagnesian silicates (ferro = iron) and are typically green, dark gray, or black in color due to the absorption of visible light by the iron and magnesium atoms. On the right side of Bowen’s reaction plagioclase crystallizes over a large temperature interval and represents a continuous series of crystallization even though its composition changes from calcium (Ca) rich to sodium (Na) rich. As the magma temperature drops and plagioclase first starts to crystallize (form), it will take in the calcium atoms into the crystal structure, but as magma temperatures continue to drop, plagioclase takes in sodium atoms preferentially. As a result, the higher temperature calcium-rich plagioclase is dark gray, in color due to the high calcium content (anorthite, or labradorite if also iridescent). The lower temperature sodium-rich plagioclase is white or lighter gray due to the high sodium content (albite).
Mineral name | % Albite (Na-rich) | % Anorthite (Ca-rich) |
---|---|---|
Albite | 100-90 | 0-10 |
Oligoclase | 90-70 | 10-30 |
Andesine | 70-50 | 30-50 |
Labradorite | 50-30 | 50-70 |
Bytownite | 30-10 | 70-90 |
Anorthite | 10-0 | 90-100 |
Finally, at the bottom of Bowen’s Reaction Series, we see three more minerals form as temperatures continue to drop. These minerals (potassium feldspar, muscovite, and quartz) are considered to be “low temperature minerals”, as they are the last to form or crystallize during cooling; conversely these three minerals are the first to melt as a rock is heated. The removal of iron and magnesium from the magma (minerals of the discontinuous series) results in the formation of these last three minerals and magma that is deficient in these chemical elements. Oftentimes, these minerals are referred to as the nonferromagnesian silicates, because they lack iron and magnesium and are considerably lighter in color; for example, potassium-rich feldspar (also known as K-feldspar or K-spar) can be pink, white, or beige in color.
Potassium Feldspar (K-feldspar) Group Minerals | |
Mineral name | Chemical formula |
Sanidine | KAlSi3O8 |
Orthoclase | |
Microcline |
The reference to mineral color is necessary, because the color of any mineral is primarily due to the chemical elements present in the mineral. Therefore, the color of an igneous rock will be dependent on the mineral content (or chemical composition) of the rock.
Igneous Rock Composition
Bowen’s Reaction Series also includes the igneous rock compositions, which are entirely dependent on the minerals that compose that rock. For example, you can expect to find abundant olivine, and maybe a little pyroxene and a little Ca-rich plagioclase, in an ultramafic rock called peridotite or komatiite. Pyroxene, plagioclase, amphibole, and possibly some olivine may be present in a mafic rock such as gabbro or basalt. You would expect to see quartz, muscovite, potassium feldspar, and maybe a little biotite and Na-rich plagioclase in a felsic (or silicic) rock such as granite or rhyolite.
The classification of an igneous rock depends partly on the minerals that may be present in the rock. Since the minerals have certain colors due to their chemical makeup, then the rocks must also have certain colors. For example, a rock composed mostly of olivine will be green in color (like Mountain Dew) due to olivine’s green color; such a rock would be considered to have an ultramafic composition. A rock that has a large amount of ferromagnesian minerals in it will be dark-colored, because the ferromagnesian minerals (besides olivine) tend to be dark colored. An igneous rock that is dark in color is referred to as having a mafic composition (“ma-” comes from magnesium, and “fic” from ferric iron). An igneous rock with a large amount of nonferromagnesian minerals will be lighter in color; these are termed silicic or felsic in composition (“fel” from feldspar, and “sic” from silica-rich quartz). A rock with a composition of intermediate, will fall between mafic and felsic. Using color, we’ll be able to properly identify any crystalline igneous rock (i.e. a rock contains crystals or minerals). Non-crystalline igneous rocks require a bit more information. As previously mentioned, classifying rocks into one of the igneous rock compositions (ultramafic, mafic, intermediate, and felsic) depends on the minerals that each rock contains.
An easy method of determining igneous rock composition is through determining the percentage of dark-colored minerals in the rock, without trying to identify the actual minerals present; this method of classification relies on a color index (CI), where the term mafic refers to any dark gray, black or green colored mineral. Igneous rocks with 0-15% dark colored minerals (or 0-15% CI) are felsic. Igneous rocks with 46-85% CI are mafic. Igneous rocks with over 85% CI are considered ultramafic. This means that any rock with between 16-45% CI has an intermediate composition.
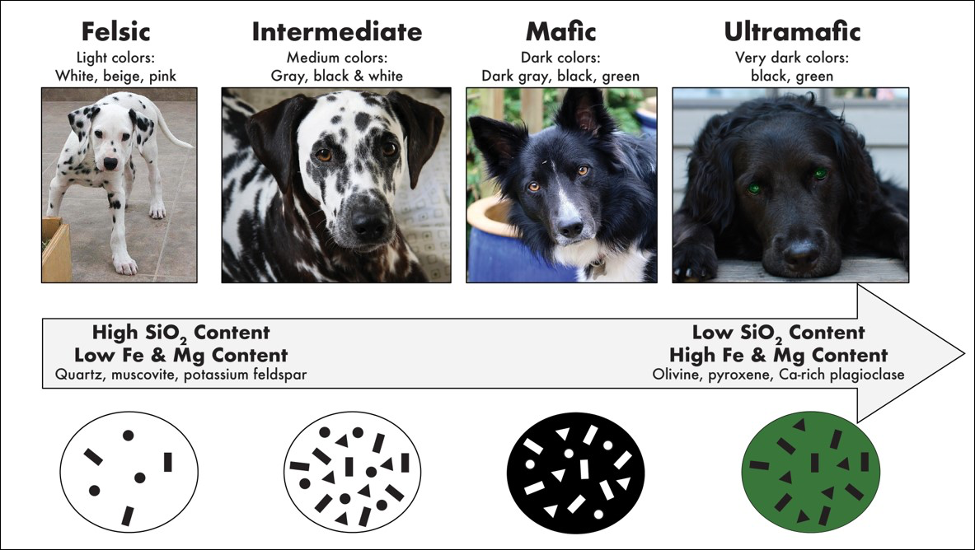
Estimating the percentage of dark-colored minerals is only possible if the minerals are large enough to see. In general, we can distinguish a mafic rock by its dark-colored appearance and a felsic rock by its light-colored appearance. An intermediate rock will be somewhat lighter than a mafic rock, yet darker than a felsic rock. Finally, an ultramafic rock is typically green in color, due to the large amount of olivine or pyroxene in the rock. Rocks that contain minerals that are too small to be seen can still be distinguished between ultramafic, mafic, intermediate and felsic by their overall color (Table 7.3).
Color Index (CI) | ||||
Composition | % of mafic minerals | Overall color | Aphanitic (extrusive) | Phaneritic (intrusive) |
Felsic | 0-15 | Light - pink, white, beige |
Rhyolite Trachyte |
Granite Syenite Monzonite |
Intermediate | 16-45 | Gray, green |
Dacite Andesite |
Granodiorite Diorite |
Mafic | 46-85 | Dark - black, green, grey | Basalt | Gabbro |
Ultramafic | >85 | Green (olivine) | Komatiite | Peridotite |
Texture
What Happens to a Melt As It Cools?
As a melt cools, minerals crystallize and glasses can develop. In general, melts will cool slowly, over millennia to millions of years (beyond a human lifespan), or rapidly, in seconds to decades (within a human lifespan). There are a number of factors that dictate the cooling rate of a melt, but the major control is the cooling environment or realm of the melt. Does the melt cool intrusively, as magma? Or does the melt cool extrusively, as lava? A melt that cools in the intrusive, or plutonic, realm cools slowly over millennia to millions of years. A melt that cools in the extrusive, or volcanic, realm cools rapidly, in seconds to decades. The extrusive realm is typically associated with volcanics, and if humans are present they may witness the eruption. The cooling realm is typically the driving factor of the rock’s texture. Generally, rocks that cool intrusively have visible crystals (minerals), whereas rocks that cool extrusively have microscopically small crystals (minerals) or contain glass. In addition to the cooling realm, the size and shape of the melt will also dictate the overall cooling rate (Figure 7.7).
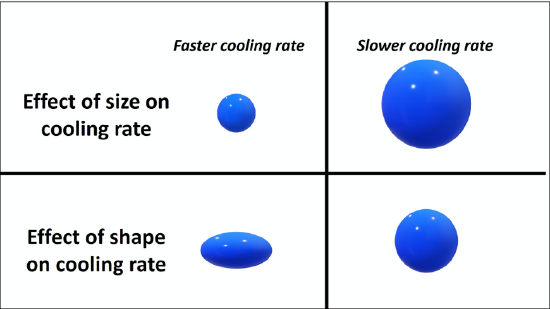
The end product of a cooling melt is an igneous rock. This rock may contain minerals, glasses, clasts, or some combination of those materials.
The classification of igneous rocks is based not just on composition, but also on texture. Recall a rocks’ texture refers to the size and arrangement of minerals, glass, fragmented material, or vesicles (holes).
Igneous Rock Textures
Crystals form while the magma is cooling. Therefore, the size of the crystals is related to the cooling process. Each mineral derives its chemical composition directly from the magma and has a certain temperature interval during which that particular mineral can form. The chemical elements that become part of the mineral must migrate from the liquid magma to link or bond with other elements in a certain way to form the crystal structure that is unique for that mineral. Whether the magma’s temperature drops quickly or slowly affects the time necessary for the migration of the chemical elements to form a mineral crystal.
When magma cools slowly, there is plenty of time for the migration of the needed chemical elements to form a certain mineral; that particular mineral can become quite large in size, large enough for a person to see without the aid of a microscope. As a result, this igneous rock with its visible minerals is said to have a phaneritic texture (phaneros = visible) (Figure 7.8). Granite, diorite, and gabbro are all phaneritic igneous rocks with different compositions (felsic, intermediate, and mafic, respectively).
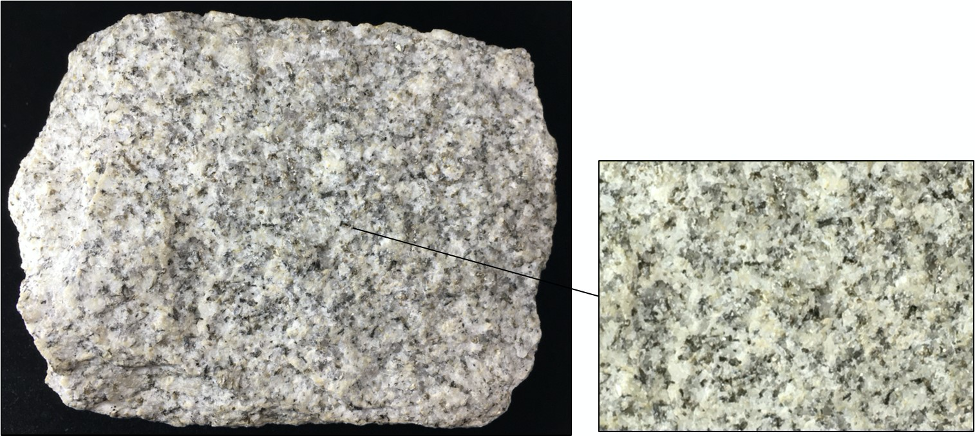
If the rock contains crystals that are larger than 1 cm (roughly the size of your thumbnail), throughout the entire rock, the textural term is pegmatitic that forms a rock called pegmatite (Figure 7.9).
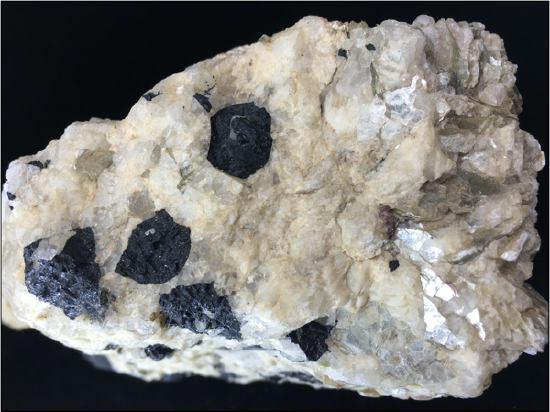
Magma that cools relatively quickly has less time for the migration of the chemical elements to form a mineral, and as a result the minerals will not have time to form large crystals. Therefore, many small crystals of a particular mineral will form in the magma. Igneous rocks that are composed of crystals too small to see (unless you have a microscope) are called aphanitic igneous rocks (Figure 7.10).
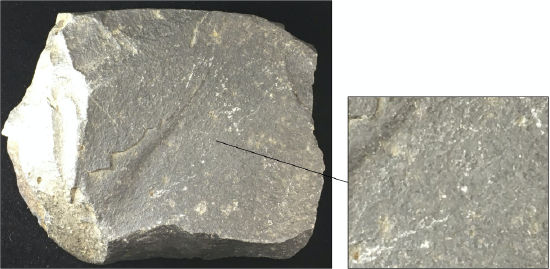
Basalt, andesite, and rhyolite are all aphanitic igneous rocks with different compositions (mafic, intermediate, and felsic, respectively). It is important to note that basalt and gabbro both have the same composition – mafic – but one rock represents a lava that cooled fast (basalt), and the other represents a mafic magma that cooled slowly (gabbro). The same can be said for the other rock compositions: the felsic rocks rhyolite and granite have identical compositions, but one cooled fast (rhyolite) and the other cooled slowly (granite). The intermediate rocks diorite and andesite also represent melts that cooled slower or more rapidly, respectively.
Sometimes there are some visible crystals in an otherwise aphanitic rock, such as the andesite shown; this texture is referred to as porphyritic (or more accurately porphyritic-aphanitic; Figure 7.11). Two different crystal sizes within the same igneous rock indicates that the cooling rate was complex, and there may be multiple levels of cooling represented. The larger crystals are called phenocrysts and the smaller crystals are called the groundmass. While the magma was cooling slowly, larger crystals can form. If the magma moves upward and erupts from a volcano, the remaining lava crystallizes quickly around the already formed phenocrysts, to form the small-size crystals in the groundmass. A phaneritic rock can also be referred to as a porphyritic-phaneritic if it contains some very large crystals (phenocrysts), in addition to the other smaller, but still visible crystals in the groundmass.
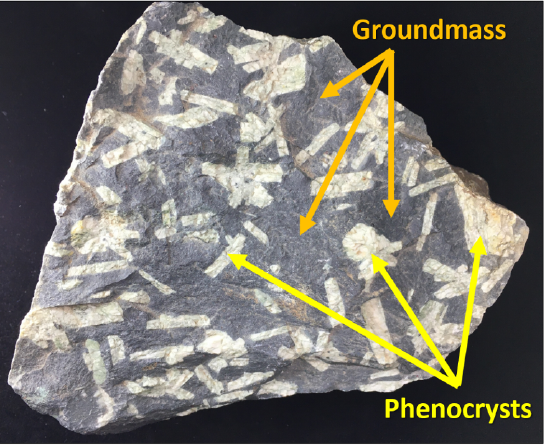
Sometimes the melt cools so quickly that there isn’t time to form any minerals, as the chemical elements in the magma do not have time to migrate into any crystal structure. When this happens, the magma becomes a dense glass called obsidian. By definition, glass is a chaotic arrangement of the chemical elements, and therefore is not considered to be a mineral; igneous rocks composed primarily of glass are said to have a glassy texture (Figure 7.12). The identification of a glassy rock such as obsidian is easy once you recall the properties of glass: any thick glass pane or a glass bottle that is broken will have a smooth, curve-shaped pattern on the broken edge called conchoidal fracture. Even though obsidian is naturally occurring, and not synthetic (human-made), it still breaks in the same way as glass you are likely familiar with. Obsidian appears quite dark in color because light has difficulty passing through the dense glass; however, chemically it is mostly silicon and oxygen and therefore more felsic in composition.
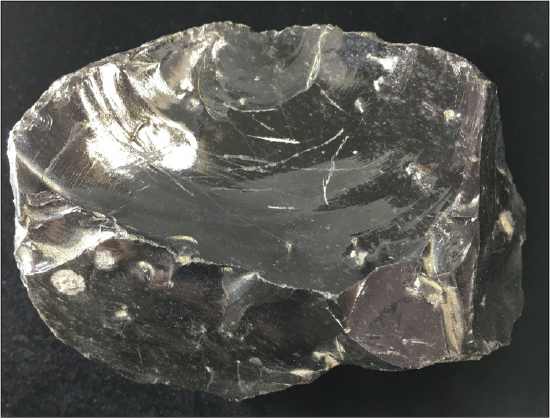
Figure 7.12: A glassy igneous rock.
Another igneous rock that is also composed primarily of glass is called pumice, which will actually float on water due to its low density. The glass in this rock has been aerated during a volcanic eruption. This aeration produces a texture referred to as vesicular or porous, and the rock typically has many holes (imagine freezing the foamy head on root beer) (Figure 7.13). Pumice can display a wide range of colors but typically has a composition between felsic to intermediate. Scoria, the other vesicular rock, will not float on water. This is because scoria contains an abundance of iron and magnesium, resulting in a dark gray, black or red color, representing a more mafic composition.
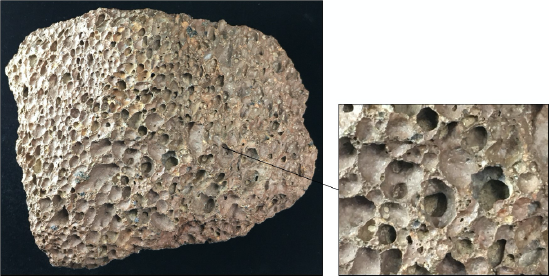
The final texture is unique in that the rock contains clasts, fragments, or pieces of pre-existing rock that were violently produced during an explosive, gas-rich, volcanic eruption. This texture is termed pyroclastic or fragmental (Figure 7.14) and has a distinct gritty or grainy feel.
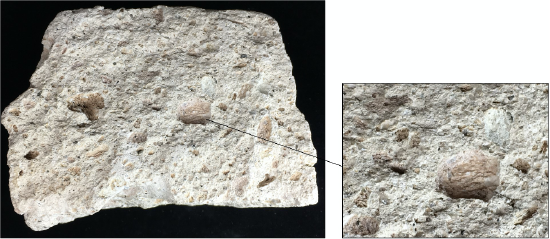
The different crystal sizes and presence or absence of glass in an igneous rock is primarily controlled by the rate of magma cooling. Generally, rocks that cool intrusively have visible crystals (minerals), whereas rocks that cool extrusively have small crystals (minerals) or contain no crystals, instead exhibiting glasses, holes, or clasts. Recall, magmas that cool below the surface in the intrusive or plutonic realm cool slowly over millennia to millions of years, because the rock surrounding the magma acts as an insulator (similar to a coffee thermos). Lavas that cool at the surface, in the extrusive or volcanic realm, cool rapidly, in seconds to decades.
Texture | Composition | |||
Felsic High SiO2 Low Fe & Mg |
Intermediate | Mafic |
Ultramafic Low SiO2 High Fe & Mg |
|
Minerals present (crystalline rocks only) |
Quartz, Potassium feldspar, Na-rich plagioclase feldspar | Na-rich plagioclase feldspar, Amphibole | Ca-Plagioclase feldspar, Pyroxene | Olivine, Pyroxene, Ca-Plagioclase feldspar |
Pegmatitic* | Granite pegmatite | -- | Gabbro pegmatite | -- |
Phaneritic* |
Granite Syenite Monzonite |
Diorite | Gabbro | Peridotite |
Porphyritic*^ |
Granite porphyry Rhyolite porphyry |
Andesite porphyry | Basalt porphyry | -- |
Aphanitic^ |
Rhyolite Trachyte |
Andesite Dacite |
Basalt | Komatiite |
Glassy^ | Obsidian | -- | -- | |
Vesicular^ | Pumice (floats on water) |
Scoria (many holes; light heft) Vesicular basalt (few holes) |
-- | |
Pyroclastic^ (Fragmental) |
Volcanic Tuff (fine grained, <4 mm) | -- | -- | |
Rhyolite tuff Dacite tuff |
Andesite tuff | |||
Volcanic Breccia (coarse grained, >4 mm) | -- | -- | ||
*intrusive ^extrusive *^complex cooling history |
Intrusive Igneous Features
Once the magma has completely crystallized, an intrusive igneous body is formed that can be generally called a pluton regardless of its shape or size. Sometimes these plutons are exposed at the Earth’s surface where direct observation of the rock is possible. They are composed of minerals that are large enough to see without the aid of a microscope. This is why any igneous rock that has visible crystals throughout (phaneritic, porphyritic-phaneritic, or pegmatitic) can be referred to as a plutonic rock.

A batholith is a large area (>100 square km) that consists of numerous types of plutons. Here in California, there are many famous batholith examples including the Sierra Nevada batholith, visible in Yosemite National Park (Figure 7.15), Peninsular Range batholith, visible around the San Diego area and south into Baja California, and the Klamath Batholith, visible in northern California along the border with Oregon.
Sills and Dikes are tabular bodies of magma that intrude into a fracture. Sills follow bedding planes, whereas dikes cross-cut beds (Figure 7.16). Tabular (sheet-like) plutons are distinguished on the basis of whether or not they are concordant with (parallel to) existing layering (e.g., sedimentary bedding or metamorphic foliation) in the country rock. A sill is concordant with existing layering, and a dike is discordant. If the country rock has no bedding or foliation, then any tabular body within it is a dike. Note that the sill-versus-dike designation is not determined simply by the orientation of the feature. A dike can be horizontal and a sill can be vertical (if the bedding is vertical). A laccolith is a sill-like body that has expanded upward by deforming the overlying rock (Figure 7.16). A stock is a discordant (cuts across other layers), small, balloon-shaped intrusive rock body (< 100 square km).
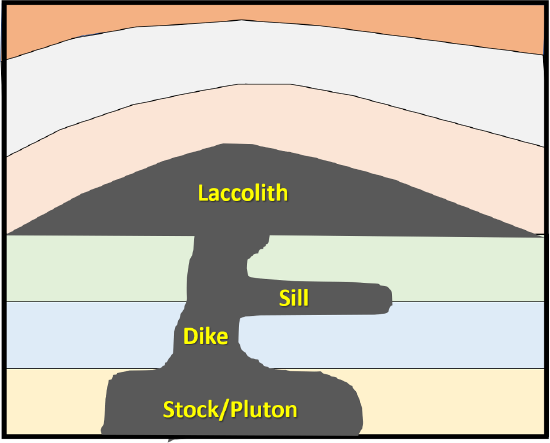
Figure 7.16: Intrusive igneous features.
Attributions
-
Figure 7.1: “The Rock Cycle” (CC-BY 4.0; Emily Haddad, own work)
-
Figure 7.2: Derivative of “Partial Melting Asthenosphere EN” (CC-BY-SA 3.0; Woudloper, via https://commons.wikimedia.org/wiki/F...osphere_EN.svg) by Emily Haddad
-
Figure 7.3: “Decompression Melting” (CC-BY 4.0; Chloe Branciforte, own work)
-
Figure 7.4: “Addition of Volatiles” (CC-BY 4.0; Chloe Branciforte, own work)
-
Figure 7.5: “Bowen’s Reaction Series” (CC-BY 4.0; Chloe Branciforte, own work)
-
Table 7.1: “Plagioclase Group Minerals” (CC-BY 4.0; Chloe Branciforte, own work)
-
Table 7.2: “Potassium Feldspar Group Minerals” (CC-BY 4.0; Chloe Branciforte, own work)
-
Figure 7.6: Derivative of (“Dalmatian Puppy” (CC-BY-SA 3.0; Carlos Estrada Dejo via Wikimedia Commons) and “Angies Bettellick” (CC-BY 2.0; Maja Dumat via Flickr) and “Border Collie, Shadow, Black & White Coat, Ireland” (CC-BY-SA 3.0; Jimbobrimbaud via Wikipedia) and “Reclining Black Dog” (CC-BY 2.0; Joe Parks via Wikimedia Commons)) by Emily Haddad and Chloe Branciforte.
-
Table 7.3: “Color Index” (CC-BY 4.0; Chloe Branciforte, own work)
-
Figure 7.7: “Cooling Rate” (CC-BY 4.0; Chloe Branciforte, own work)
-
Figure 7.8: “Phaneritic Texture” (CC-BY 4.0; Chloe Branciforte, own work)
-
Figure 7.9: “Pegmatite Texture” (CC-BY 4.0; Chloe Branciforte, own work)
-
Figure 7.10: “Aphanitic Texture” (CC-BY 4.0; Chloe Branciforte, own work)
-
Figure 7.11: “Porphyritic Texture” (CC-BY 4.0; Chloe Branciforte, own work)
-
Figure 7.12: “Glassy Texture” (CC-BY 4.0; Chloe Branciforte, own work)
-
Figure 7.13: “Vesicular Texture” (CC-BY 4.0; Chloe Branciforte, own work)
-
Figure 7.14: “Fragmental Texture” (CC-BY 4.0; Chloe Branciforte, own work)
-
Table 7.4: “Igneous Rock Chart” (CC-BY 4.0; Chloe Branciforte, own work)
-
Figure 7.15: “Sierra Nevada Batholith” (CC-BY 2.0; James St. John via Flickr)
-
Figure 7.16: “Intrusive Igneous Features” (CC-BY 4.0; Chloe Branciforte, own work)