4.1: Front Matter
- Page ID
- 14523
\( \newcommand{\vecs}[1]{\overset { \scriptstyle \rightharpoonup} {\mathbf{#1}} } \)
\( \newcommand{\vecd}[1]{\overset{-\!-\!\rightharpoonup}{\vphantom{a}\smash {#1}}} \)
\( \newcommand{\id}{\mathrm{id}}\) \( \newcommand{\Span}{\mathrm{span}}\)
( \newcommand{\kernel}{\mathrm{null}\,}\) \( \newcommand{\range}{\mathrm{range}\,}\)
\( \newcommand{\RealPart}{\mathrm{Re}}\) \( \newcommand{\ImaginaryPart}{\mathrm{Im}}\)
\( \newcommand{\Argument}{\mathrm{Arg}}\) \( \newcommand{\norm}[1]{\| #1 \|}\)
\( \newcommand{\inner}[2]{\langle #1, #2 \rangle}\)
\( \newcommand{\Span}{\mathrm{span}}\)
\( \newcommand{\id}{\mathrm{id}}\)
\( \newcommand{\Span}{\mathrm{span}}\)
\( \newcommand{\kernel}{\mathrm{null}\,}\)
\( \newcommand{\range}{\mathrm{range}\,}\)
\( \newcommand{\RealPart}{\mathrm{Re}}\)
\( \newcommand{\ImaginaryPart}{\mathrm{Im}}\)
\( \newcommand{\Argument}{\mathrm{Arg}}\)
\( \newcommand{\norm}[1]{\| #1 \|}\)
\( \newcommand{\inner}[2]{\langle #1, #2 \rangle}\)
\( \newcommand{\Span}{\mathrm{span}}\) \( \newcommand{\AA}{\unicode[.8,0]{x212B}}\)
\( \newcommand{\vectorA}[1]{\vec{#1}} % arrow\)
\( \newcommand{\vectorAt}[1]{\vec{\text{#1}}} % arrow\)
\( \newcommand{\vectorB}[1]{\overset { \scriptstyle \rightharpoonup} {\mathbf{#1}} } \)
\( \newcommand{\vectorC}[1]{\textbf{#1}} \)
\( \newcommand{\vectorD}[1]{\overrightarrow{#1}} \)
\( \newcommand{\vectorDt}[1]{\overrightarrow{\text{#1}}} \)
\( \newcommand{\vectE}[1]{\overset{-\!-\!\rightharpoonup}{\vphantom{a}\smash{\mathbf {#1}}}} \)
\( \newcommand{\vecs}[1]{\overset { \scriptstyle \rightharpoonup} {\mathbf{#1}} } \)
\( \newcommand{\vecd}[1]{\overset{-\!-\!\rightharpoonup}{\vphantom{a}\smash {#1}}} \)
\(\newcommand{\avec}{\mathbf a}\) \(\newcommand{\bvec}{\mathbf b}\) \(\newcommand{\cvec}{\mathbf c}\) \(\newcommand{\dvec}{\mathbf d}\) \(\newcommand{\dtil}{\widetilde{\mathbf d}}\) \(\newcommand{\evec}{\mathbf e}\) \(\newcommand{\fvec}{\mathbf f}\) \(\newcommand{\nvec}{\mathbf n}\) \(\newcommand{\pvec}{\mathbf p}\) \(\newcommand{\qvec}{\mathbf q}\) \(\newcommand{\svec}{\mathbf s}\) \(\newcommand{\tvec}{\mathbf t}\) \(\newcommand{\uvec}{\mathbf u}\) \(\newcommand{\vvec}{\mathbf v}\) \(\newcommand{\wvec}{\mathbf w}\) \(\newcommand{\xvec}{\mathbf x}\) \(\newcommand{\yvec}{\mathbf y}\) \(\newcommand{\zvec}{\mathbf z}\) \(\newcommand{\rvec}{\mathbf r}\) \(\newcommand{\mvec}{\mathbf m}\) \(\newcommand{\zerovec}{\mathbf 0}\) \(\newcommand{\onevec}{\mathbf 1}\) \(\newcommand{\real}{\mathbb R}\) \(\newcommand{\twovec}[2]{\left[\begin{array}{r}#1 \\ #2 \end{array}\right]}\) \(\newcommand{\ctwovec}[2]{\left[\begin{array}{c}#1 \\ #2 \end{array}\right]}\) \(\newcommand{\threevec}[3]{\left[\begin{array}{r}#1 \\ #2 \\ #3 \end{array}\right]}\) \(\newcommand{\cthreevec}[3]{\left[\begin{array}{c}#1 \\ #2 \\ #3 \end{array}\right]}\) \(\newcommand{\fourvec}[4]{\left[\begin{array}{r}#1 \\ #2 \\ #3 \\ #4 \end{array}\right]}\) \(\newcommand{\cfourvec}[4]{\left[\begin{array}{c}#1 \\ #2 \\ #3 \\ #4 \end{array}\right]}\) \(\newcommand{\fivevec}[5]{\left[\begin{array}{r}#1 \\ #2 \\ #3 \\ #4 \\ #5 \\ \end{array}\right]}\) \(\newcommand{\cfivevec}[5]{\left[\begin{array}{c}#1 \\ #2 \\ #3 \\ #4 \\ #5 \\ \end{array}\right]}\) \(\newcommand{\mattwo}[4]{\left[\begin{array}{rr}#1 \amp #2 \\ #3 \amp #4 \\ \end{array}\right]}\) \(\newcommand{\laspan}[1]{\text{Span}\{#1\}}\) \(\newcommand{\bcal}{\cal B}\) \(\newcommand{\ccal}{\cal C}\) \(\newcommand{\scal}{\cal S}\) \(\newcommand{\wcal}{\cal W}\) \(\newcommand{\ecal}{\cal E}\) \(\newcommand{\coords}[2]{\left\{#1\right\}_{#2}}\) \(\newcommand{\gray}[1]{\color{gray}{#1}}\) \(\newcommand{\lgray}[1]{\color{lightgray}{#1}}\) \(\newcommand{\rank}{\operatorname{rank}}\) \(\newcommand{\row}{\text{Row}}\) \(\newcommand{\col}{\text{Col}}\) \(\renewcommand{\row}{\text{Row}}\) \(\newcommand{\nul}{\text{Nul}}\) \(\newcommand{\var}{\text{Var}}\) \(\newcommand{\corr}{\text{corr}}\) \(\newcommand{\len}[1]{\left|#1\right|}\) \(\newcommand{\bbar}{\overline{\bvec}}\) \(\newcommand{\bhat}{\widehat{\bvec}}\) \(\newcommand{\bperp}{\bvec^\perp}\) \(\newcommand{\xhat}{\widehat{\xvec}}\) \(\newcommand{\vhat}{\widehat{\vvec}}\) \(\newcommand{\uhat}{\widehat{\uvec}}\) \(\newcommand{\what}{\widehat{\wvec}}\) \(\newcommand{\Sighat}{\widehat{\Sigma}}\) \(\newcommand{\lt}{<}\) \(\newcommand{\gt}{>}\) \(\newcommand{\amp}{&}\) \(\definecolor{fillinmathshade}{gray}{0.9}\)What’s Under Our Feet?
Pop culture and Hollywood have journeyed to the center of the Earth several times; however, humans have never actually been there. We are not even able to see directly into the Earth’s interior! Instead, geologists use evidence from drilling, proxies like moon rocks and meteorites, samples of the Earth’s interior expelled by volcanoes (like diamonds and peridotite), seismic activity, and computer modeling to learn more about the composition and structure of Earth’s interior.
Knowledge of the Earth’s interior is the basis for geology and helps us understand the many processes operating inside and at the Earth’s surface, notably plate tectonics. In this section, we will see how the Earth is structured, what its physical characteristics are, and how the internal structure of the Earth impacts us living on the surface.
Seismologists, geophysicists, volcanologists, and other disciplines may all study the internal Earth. Each branch uniquely investigates Earth’s internal structure and the impacts that structure has on its internal and external workings. Like other geoscientists, interdisciplinary collaboration is common, with a strong influence from both math and technology sectors. Many of these geoscientists are employed by universities where they teach and/or do research, and state and federal agencies, including geological surveys like the California Geological Survey or United State Geological Survey (USGS). Additional career pathways are available in the private sector, including in mining and natural resource extraction. Many of these career options require a college degree and postgraduate work. If you are interested in becoming a deep earth scientist, talk to your geology instructor for advice. We recommend completing as many math and science courses as possible (chemistry is incredibly important for mineralogy). Also, visit National Parks, CA State Parks, museums, gem & mineral shows, or join a local rock and mineral club. Typically, natural history museums will have wonderful displays of rocks, including those from your local region. Here in California, there are a number of large collections, including the San Diego Natural History Museum, Natural History Museum of Los Angeles County, Santa Barbara Museum of Natural History, and Kimball Natural History Museum. Many colleges and universities also have their own collections/museums.
Before we can address plate tectonics, we must consider Earth’s layered interior. At first glance, we can identify three major regions which are distinct based on their overall composition: the core, mantle, and crust. However, more layers are visible if we also consider mechanical properties of the layers. By examining these properties, five major layers are identified, the lithosphere (upper mantle and crust), asthenosphere, lower mantle, outer core, and inner core. For a visual journey through the interior of the Earth, visit the BBC's Journey to the Center of the Earth or watch this video and take a trip to the Earth’s core.
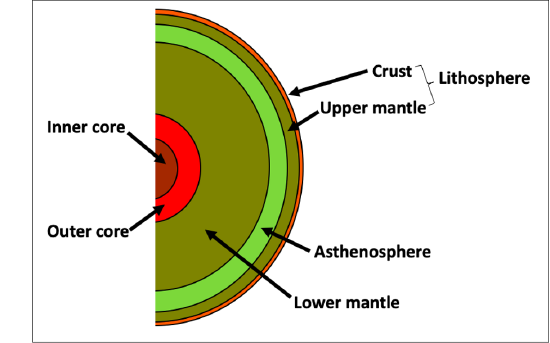
Properties | Lithosphere | Asthenosphere | Lower Mantle | Outer Core | Inner Core |
---|---|---|---|---|---|
Physical behavior | Solid, rigid, brittle | Solid, plastic (ductile) | Solid, rigid, brittle | Liquid | Solid, rigid, not brittle |
Overall Thickness (mi) | 62 | 115 | 1800 | 1367 | 776 |
Overall Thickness (km) | 100 | 185 | 2897 | 2200 | 1249 |
The lithosphere, which is composed of the crust and upper mantle, is about 100 km (~62 mi) thick and behaves as a brittle, rigid solid (Figure 4.2). This brittleness causes breaks (earthquakes) when stresses are applied. The crust is very thin relative to the radius of the planet; however, there are two distinct types: 1) oceanic crust, which is composed mainly of mafic igneous rocks like basalt and gabbro and 2) continental crust, which is composed mainly of the felsic igneous rock, granite. The two crustal types are very different and their density differences are significant to the mechanics of plate tectonics. Granite is much less dense than basalt, which results in a buoyant continental crust that sits higher on the underlying mantle. The denser and less buoyant oceanic crust sinks further into the underlying mantle, forming basins, many of which are filled with the Earth’s oceans. Table 4.2 highlights crustal differences in more detail.
Crustal type | Average thickness | Density | Composition | Dominant rock type |
---|---|---|---|---|
Oceanic | 7 km / 4 mi | 3.0 g/cm3 | Mafic | Basalt and gabbro |
Continental | 40 km / 25 mi | 2.7 g/cm3 | Felsic | Granite |
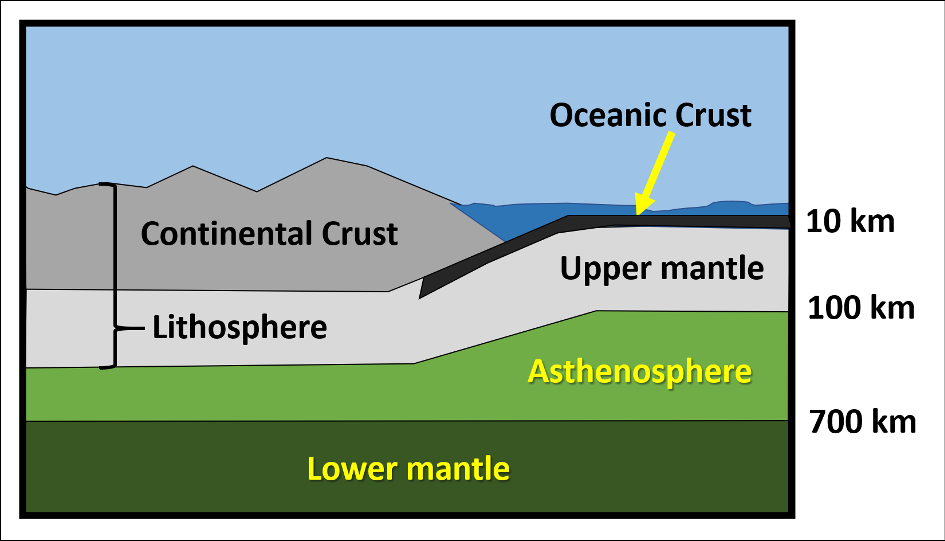
Figure 4.2: Components of the lithosphere, including the continental crust, oceanic crust, and upper mantle.
The mantle is made of hot, solid rock, much of which is composed of silicate minerals such as olivine. The asthenosphere, a region of the mantle that is plastic and capable of flow, is likely composed mainly of the ultramafic rock peridotite. The lower mantle (mesosphere) is more rigid and rocky and is composed of different silicate minerals, such as bridgmanite.
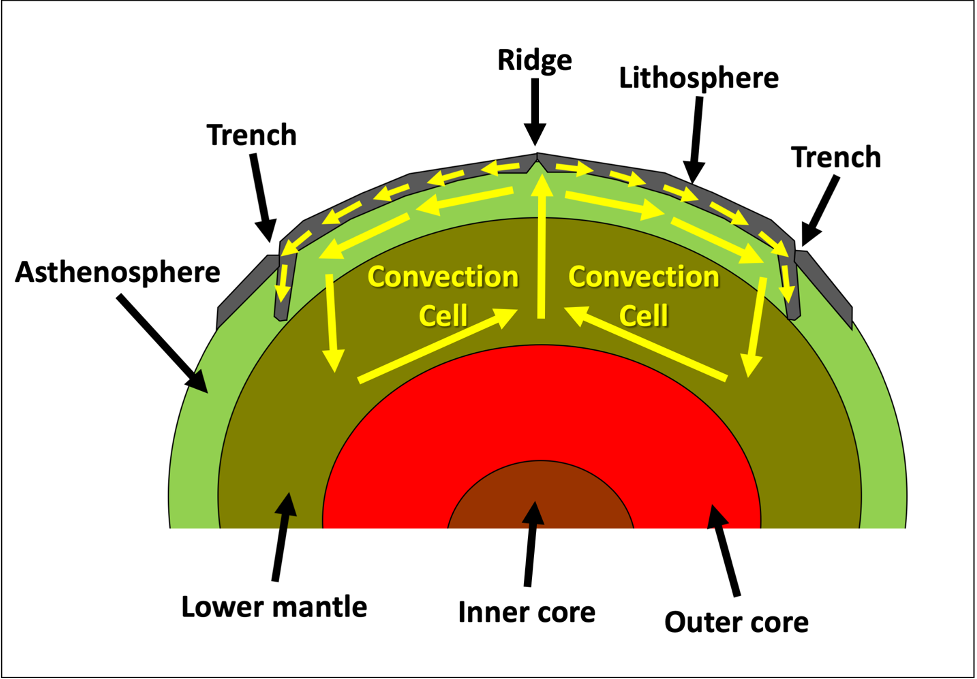
Figure 4.3: The convection cells of the mantle
Convection currents, important to the mechanics of plate tectonics, develop as lower mantle materials near the core heat up. These mantle convection cells occur as particles in the rock begin to move more rapidly, decrease in density, rise toward the surface of the Earth. When the warm material reaches the surface, it spreads horizontally and begins to cool. Eventually the material becomes cool and dense enough to sink back down into the mantle toward the core. As the material sinks back to the bottom of the mantle, the material travels horizontally and the process begins again (Figure 4.3).
At the planet’s center lies the core, which is dense and metallic. Calculations indicate that the core is about 85% iron metal with nickel metal making up most of the remaining 15%. Geologists know the core is metal because of proxies, like metallic meteorites, and from calculations of the overall density of the planet. Moving deeper into the interior of the Earth, temperature and pressure increase. Although the inner core is very hot (5200°C or 9392°F), it is solid because it is experiencing very high pressure (3.6 million atm). The pressure in the outer core is not high enough to keep it solid. Much of the heat here is produced by the breakdown of radioactive elements in the inner core.
Earthquake waves are used by geologists to “see” Earth’s internal structure, similar to how a doctor uses a CT scan to look inside your body. This imaging of the Earth’s interior by earthquake waves is seismic tomography, which highlights distinct boundaries, or discontinuities, between layers, where composition and matter changes occur. Because the inner core of the Earth is a solid metallic sphere made mostly of iron and nickel and surrounded entirely by liquid, we can visualize it as a giant ball bearing spinning in a pressurized fluid. Seismic tomography of the inner core has found evidence that it is spinning - rotating - just slightly faster than the rest of the Earth.
Earth’s magnetosphere is produced by the interaction of the rapidly rotating solid inner core and the convection and electrically-charged nature of the liquid outer core (the geodynamo). This causes instability, and every several hundred thousand to several million years, Earth’s magnetic field becomes unstable to the point that “flips”. When it “flips”, its north and south magnetic poles switch; this is called a magnetic reversal. Mathematical models suggest a reversal may take about one to several thousand years to complete.
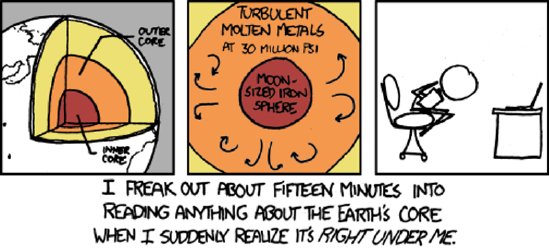
Attributions
-
Figure 4.1: “The Layered Earth” (CC-BY 4.0; Chloe Branciforte, own work)
-
Table 4.1: “Properties of Internal Earth” (CC-BY 4.0; Chloe Branciforte, own work)
-
Table 4.2: “Crustal Types” (CC-BY 4.0; Chloe Branciforte, own work)
-
Figure 4.2: “The Lithosphere” (CC-BY 4.0; Chloe Branciforte, own work)
-
Figure 4.3: “Convection” (CC-BY 4.0; Chloe Branciforte, own work)
-
Figure 4.4: “Core” (CC-BY-NC 2.5; xkcd via xkcd.com)